Active Chilled Beams Come of Age
How Chilled Beams Work
In a chilled beam, the term “induction” is used to describe the process of injecting primary air under pressure through a nozzle, which in turn entrains surrounding air at the discharge of the nozzle. The amount of air that is entrained vs. the amount of air injected is referred to as the induction ratio. It is important to note that the air pressures that cause this induction are very low so care must be taken when designing a beam (or tailoring a beam for a project) to ensure that there is as low a restriction as possible in the beam to keep the induction ratio high.
The induced air is drawn through a water coil that may either heat or cool the air before it comes in contact with the injected air discharging from the nozzle. The amount of energy transferred by the water coil is influenced by several factors, including the induced air temperature, water supply temperature, volume of induced air moving through the water coil, and amount of coil surface area.
When designing a chilled beam system, one parameter that is often used is the transfer effectiveness (watts/cfm). This is the fraction of heating or cooling energy that is provided by the water coil heat transfer (q water coil) vs. the volume of primary air.
Transfer Effectiveness = q water coil / Q primary air
The transfer effectiveness is related to the overall HVAC system effectiveness. It takes less horsepower to move thermal energy with water than it does with air, as the volume of air needed is significantly larger due to the lower amount of thermal energy it can store. The potential saving is significant, but limited by the system choices made by the designer, as well as the primary air volume requirements. To reach the point of minimal energy consumption used to move air, the primary air volume would be no more than the requirements for fresh air.
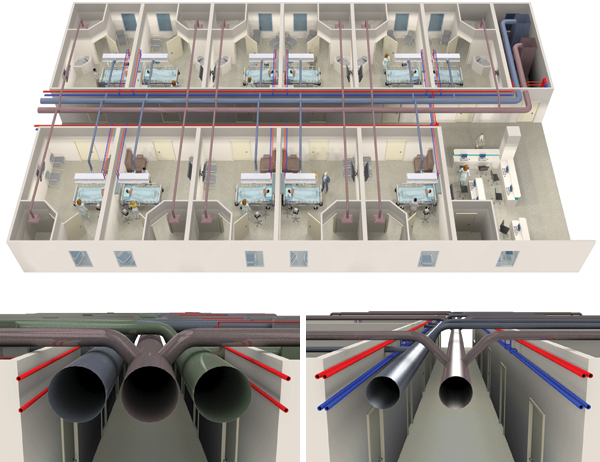
Images courtesy of Price Industries
Hospital patient room hall interstitial space for all-air HVAC system (supply, return, and exhaust) vs. hospital patient room hall interstitial space for an active chilled beam application (supply duct, exhaust duct, chilled water piping)
Minimizing primary air volume is the key to lowered HVAC brake horsepower and higher energy efficiency. Unfortunately, many designers only have experience with overhead mixing air distribution, which requires higher volumes of air, and do not feel comfortable lowering the primary air to the lowest level, leading to less than optimal energy consumption. For example, the accompanying table shows the potential air volume reductions for several different building types. The smaller air-side load fractions indicate the potential for the HVAC designer to use minimal primary air and obtain higher energy savings due to the lowered brake horsepower consumption. If the designer is able to shift the bulk of the sensible load to the water, the building total brake-horsepower has the potential to be reduced to the lowest possible amount, leading to a more efficient design. For example, for an office space, the typical ventilation (outside air) requirements is around 0.15 cfm/sf, but the thermal loads (both sensible and latent) require an all-air system to use as much as 1 cfm/sf, creating a potential for a reduction of 85 percent of the primary air volume. In a classroom, a reduction of 67 percent is possible when designing at ventilation minimums.
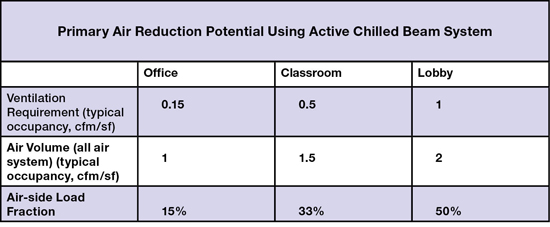
Source: ASHRAE Std 62.1 2013 and Price Industries
As a reminder, the HVAC designer needs to verify that the ventilation air is capable of handling the zone latent load.
Benefits of Reduced Primary Air Volume
Active beams can supply2 a significant portion of the sensible cooling or heating load of a building with a relatively low ventilation rate. In most commercial buildings, the ventilation rate required to condition the building can potentially be reduced by up to 85 percent of the ventilation normally required by an all-air system. A study by Weiger detailed the potential benefits of active chilled beams in a hospital setting. Chilled beam HVAC systems in non-surgical spaces such as patient rooms and office areas were found to have the potential to shorten the construction cycle and lower the cost, both initial and life cycle.3
On the scale of the overall system, the reduction in airflow will translate to a smaller air handler and smaller duct network. The reduction in system size may allow a lower floor-to-floor height as well as an increase in usable space on each floor due to a reduced footprint of the mechanical shafts. In certain building types such as healthcare facilities, reducing the congestion in the interstitial spaces will speed construction and allow for more usable floor area due to the significant reduction in the riser spaces needed. Weiger found that the cross sectional ductwork could be reduced by 75 percent with a corresponding reduction of 50 percent material ductwork cost. The riser footprint was reduced by 50 percent and savings in mechanical room footprint was estimated at 25 percent.
The reduction in riser and mechanical room footprints frees that area to be used in revenue-generating activities such as patient rooms. The reduction in riser footprint could in fact allow for an additional patient room on each floor with essentially no additional cost when compared to using a traditional all-air HVAC system. In healthcare applications, when the system is designed to provide 100 percent fresh air at code-required volumes to patient rooms, the return duct may actually not be needed as the exhaust air volume from the patient room bathroom often matches the room supply. It should also be noted that the installation of the ductwork in patient wards is complex and often requires significant coordination and stops and starts from the sheet metal contractors as they allow for other services to be installed. With less ductwork, a common result is that the mechanical system is taken off the critical path of the construction of these wards.
Energy Savings Potential
How much energy can really be saved by active chilled beams? There is no easy answer due to the many systems and possible combinations of equipment available to provide the cooling or heating water. To optimize the choice, designers must consider factors directly related to the HVAC mechanical system energy consumption including: the building geographic location; outdoor humidity levels; occupancy type/schedule; the type/source of hot/warm water; and the potential for primary air volume reduction.
Numerous buildings have incorporated air-water systems that have resulted in greater energy savings than all-air systems. The complete mechanical system supporting the air-water system must be considered, rather than simply picking an active chilled beam and expecting high energy savings. The designer has many choices. Among them:
• Buildings with a central plant with distributed chilled water are in a good position to use chilled beams. The return loop water temperature is very close to the suggested chilled beam supply water temperature. That means that return loop water could be used to provide the cooling effect which, in turn, raises the return loop water temperature and potentially increases the central plant chiller efficiency.
• Using packaged air handling equipment can increase the efficiency of the water system as the plant can be optimized to supply higher chilled water, say 55°F, rather than have to deliver very low temperatures to air handlers for dehumidification, around 42°F. As an example, a centrifugal chiller that delivers chilled water for an all-air system at 42°F may see a 30 percent improvement in the coefficient of performance, when delivering water at a temperature of 55°F.
• Designing the chiller plant to optimize economizer duty—running the cooling towers in parallel with the beam chilled water supply system will lead to an increase in free cooling hours by 25 to 50 percent in most climates.
• If the geology supports ground source heat pump systems, the water temperatures delivered to the building will be very close to the suggested supply temperatures for both heating and cooling using active chilled beams. As a system, this approach has been shown to provide high energy savings and lower operational costs.