Embracing the Timber Age
Air
Air movement across the building enclosure is caused by a pressure differential between the inner and outer enclosure surfaces. This difference can usually be attributed to a combination of wind, the stack effect, and mechanical ventilation systems.
A continuous AB is a requirement of both the 2018 International Energy Conservation Code (IECC) and the National Building Code of Canada to control airflow across the enclosure. Limiting this airflow both inward and outward, has significant performance benefits, such as reduced energy consumption for building heating and cooling, improved occupant comfort by limiting drafts, reduced risk for interstitial condensation from moisture-laden air, and minimized transfer of other undesirable substances, such as sound, smoke, fire, and pollutants.
The AB is not a single component or material, but rather requires a system of materials, components, and assemblies that provide continuous air control throughout the building enclosure. Newly manufactured CLT panels may be shown to be impermeable to air when tested in a laboratory setting. However, CLT panels will rarely remain airtight over the course of their transportation, construction, and service life due to potential dimensional changes that may cause delamination and/or cracks to form, as stated in the Canadian-based forest sector research and development firm FPInnovations’ CLT Handbook (see Figure 2-5).
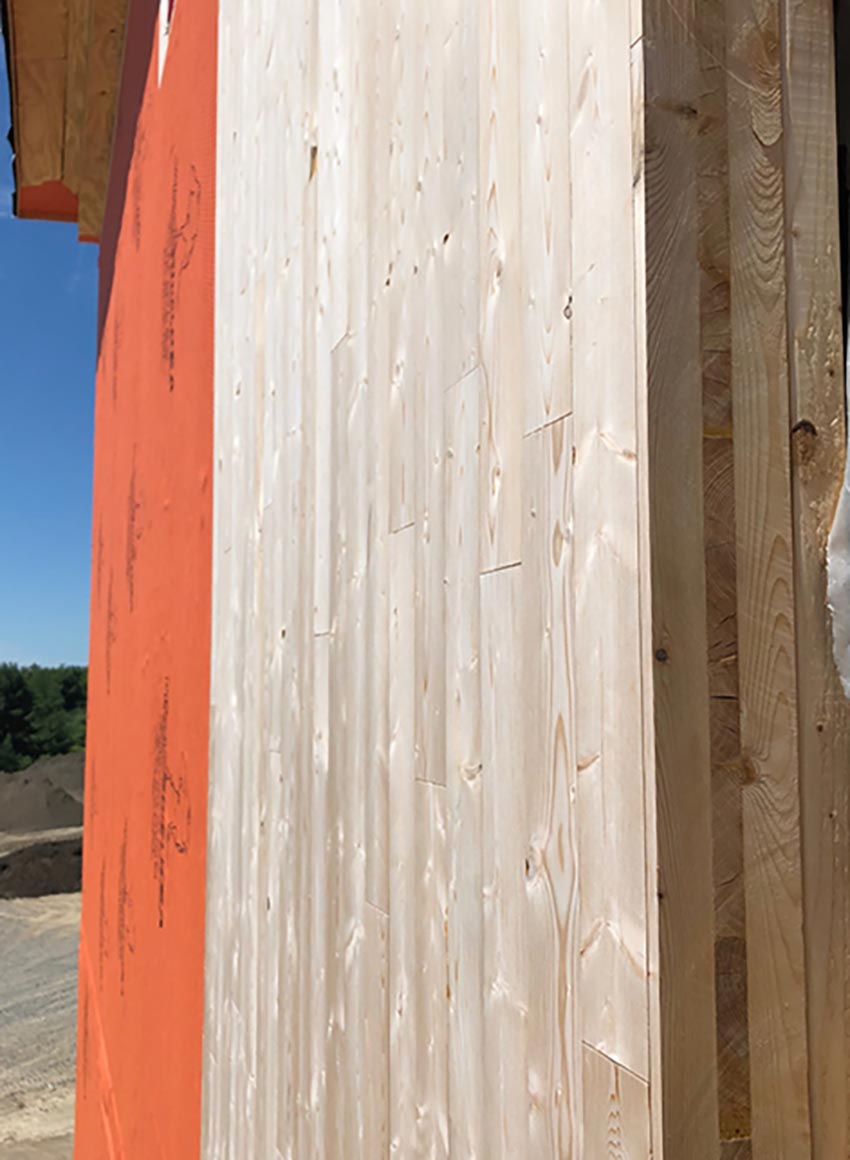
Figure 2-5
Small gaps between CLT laminations may not be completely airtight, thus requiring a separate AB membrane.
AB continuity at joints and interfaces of erected CLT panels must also be considered for a complete AB system. An AB membrane over the CLT panel or the use of flashing/tape products at these interfaces are required for the long-term airtightness of the enclosure.
For an AB to be effective, it must be impermeable to air, continuous, stiff, strong, and durable.
Heat
Heat will flow across the building enclosure when there is a temperature difference between the interior and exterior environments—the greater the temperature difference, the greater the thermal load. Thus, the heat or thermal load tends to be more influential in colder climates where the outdoor temperature dips below indoor temperatures throughout much of the year. Insulation is an essential component for energy-efficient buildings in most, if not all, North American climate zones. Selection and placement of the insulation can also improve occupant thermal comfort, provide a buffer from outdoor noise, and help manage condensation risk within the CLT enclosure.
CLT panels contribute to the assembly’s thermal performance because wood has a relatively low thermal conductivity when compared to other common structural materials such as concrete and steel. Most building codes across North America typically reference some version of the IECC, American Society of Heating, Refrigerating and Air-Conditioning Engineers (ASHRAE) 90.1, or the National Energy Code of Canada for Buildings (NECB) to set the minimum required level of insulation. Minimum insulation requirements generally fall within the R-15 to R-31 (RSI 2.6 to RSI 5.5) range for walls, and R-29 to R-48 (RSI 5.1 to RSI 8.5) for roofs. However, project-specific performance requirements may require greater levels of insulation than that required by code.
The thermal resistance of wood per inch varies based on moisture content and species, but for most commonly used North American softwood species, a thermal resistance of R-1.2 (RSI 0.21) per inch can be used, according to ASHRAE’s Handbook: Fundamentals. Thus, a five-ply, 55⁄8-inch thick CLT panel can be expected to contribute R-6.8 (RSI 1.9) to the enclosure assembly’s thermal performance. In most scenarios, the thermal resistance of the CLT panel alone will not be great enough to meet the required thermal performance of the assembly, and additional insulation will be needed. It is typically desirable to locate insulation outboard of the CLT to keep the CLT panel closer to the indoor temperature, minimizing condensation risk and limiting temperature and relative humidity fluctuations that may result in dimensional cycling of the CLT.
When a more highly conductive element, such as metal, bridges the insulation layer, it creates a path of lower resistance to heat flow. This path is commonly called a thermal bridge and will degrade the thermal performance of the insulation layer and overall assembly thermal performance. For CLT assemblies, it is common to locate the insulation exterior of the CLT, keeping the large structural connections inboard of the insulation layer. Thus, thermal bridges at CLT assemblies tend to result from a need to transfer wind loads and cladding loads back to the structure. Cladding and roof attachments/fasteners can have a significant impact on the thermal performance of the enclosure and can vary widely based on the system used. Generally, more thermally efficient options for these attachment points rely on less thermally conductive materials such as stainless steel, fiberglass, and/or intermittent attachments through the insulation, such as clips or fasteners, in lieu of continuous metal furring, according to RDH Building Science’s Cladding Attachment Solutions for Exterior Insulated Commercial Wall Assemblies. Two examples of thermally efficient cladding attachment solutions are shown in Figures 2-6 and 2-7. The attachment method will depend on the type of insulation used. Additionally, sealing may be required for some cladding attachment components where the attachments penetrates the WRB and AB membrane.
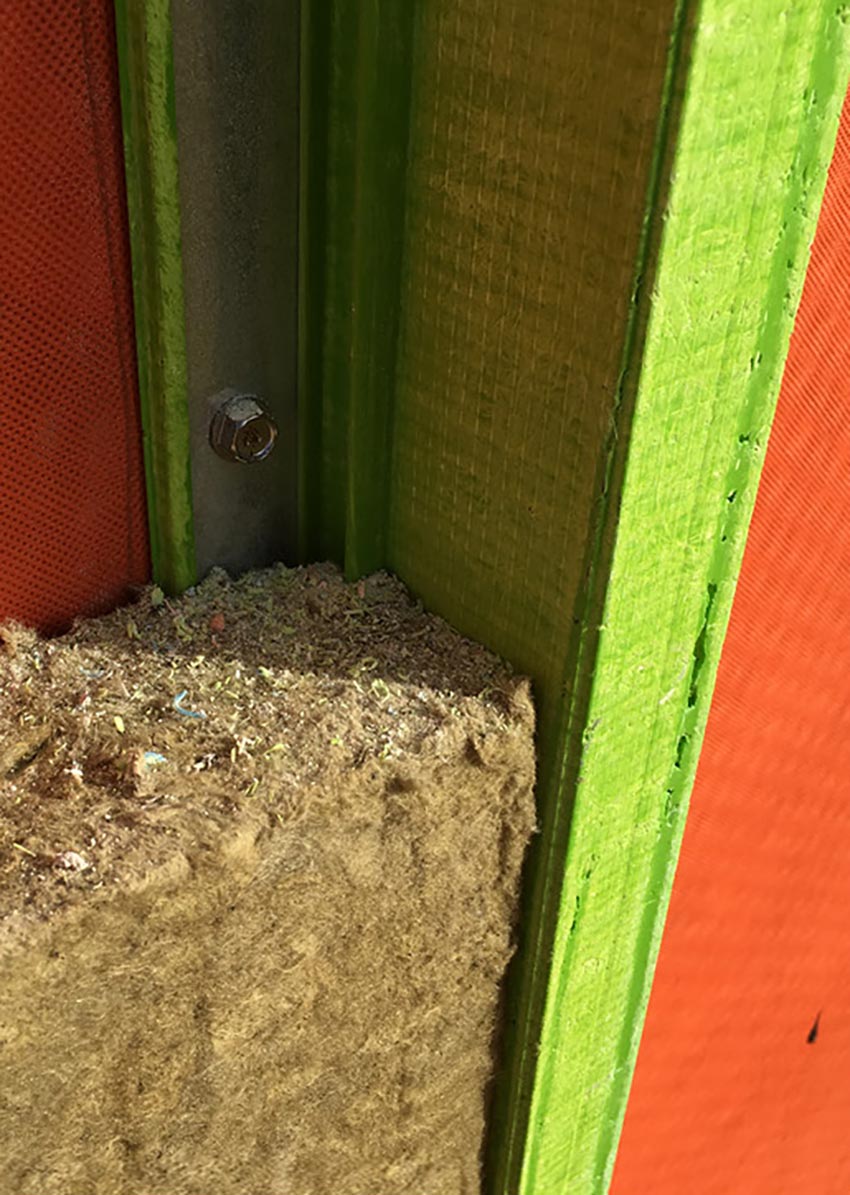
Figure 2-6
Mineral-fiber insulation is being installed between vertically oriented fiberglass girts.
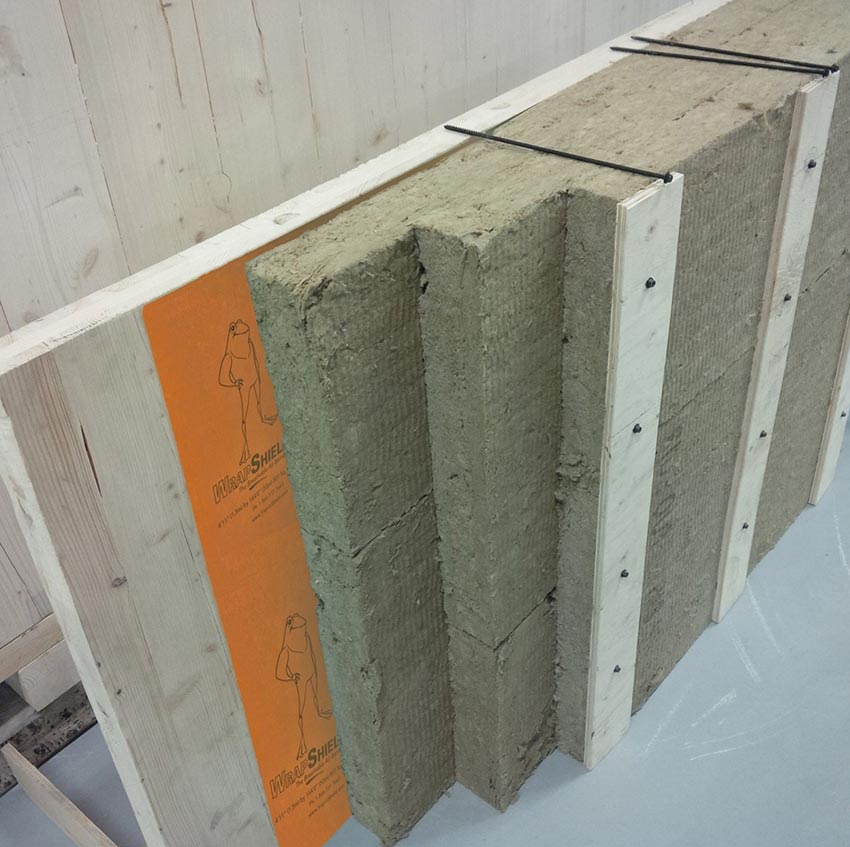
Figure 2-7
Vertical wood furring with long-screw fastener attachments and rigid mineral-fiber insulation are installed over a self-adhered WRB/AB.
The mass of the CLT panel can also impact the thermal performance of the assembly by moderating heat flow through thermal storage. Depending on climate and other building factors, this thermal storage and throttling effect may reduce both annual and peak heating and cooling requirements, particularly in warmer climates with large daily temperature variations. Building codes that reference the IECC or ASHRAE 90.1 may credit this thermal mass effect with less-stringent prescriptive insulation requirements for mass wall and floor assemblies. A CLT assembly may exceed the minimum heat capacity required by IECC/ASHRAE 90.1 to meet the definition of a mass wall or floor. However, this will largely depend on the thickness of the panel, the wood species used, and code interpretations by the local governing jurisdiction.
Vapor
Water vapor molecules diffuse through materials from areas of high concentration to low concentration (i.e., from high humidity to low humidity). The resulting “vapor drive” can be an important consideration in the design of CLT enclosures. An accumulation of water vapor in the CLT assembly can result in interstitial condensation and/or excessive moisture uptake by the CLT. In most applications, the resulting direction of the vapor drive is from the warm side to the cold side of the enclosure such that in colder weather, the direction of the vapor drive is from indoors to outdoors. It is important to consider that the direction of the vapor drive may switch throughout the year as seasons change. However, vapor diffusion through most materials is a relatively slow process. Therefore, the more prevailing environmental conditions are most often considered. Additionally, depending on the space-conditioning needs, some interior environments may not subscribe to this rule of thumb and should be carefully considered during enclosure design.
While controlling air leakage across the enclosure is an effective means of managing water vapor, providing a separate vapor retarder is often also required to moderate the diffusion of water vapor across the materials within an assembly. The 2018 International Building Code (IBC) defines three classes of vapor retarding materials. At typical indoor humidity levels, the permeance of a three-ply, 31⁄2-inch CLT panel is between 0.05 to 0.6 U.S. perms (3 ng/Pa·s·m2 to 35 ng/Pa·s·m2) and thicker CLT panels are even less permeable, corresponding to a Class 1 or 2 vapor retarder.
When insulation is located entirely outboard of the CLT panel in cold, heating-dominated climates (i.e., ASHRAE/IECC Climate Zones 4 through 8), a separate vapor retarder is likely not necessary and is often not advisable. Omitting the vapor retarder allows the panel to dry to the interior as needed from seasonal wetting or construction.
WRB and AB sheet membranes, roof underlayment sheet membranes, and most flashing membranes should be vapor permeable to allow for some seasonal drying of the CLT panel. In these scenarios, it is also beneficial to use exterior insulation that is vapor permeable to allow drying to the exterior environment and avoid trapping vapor behind the insulation, as shown in Figure 2-8.
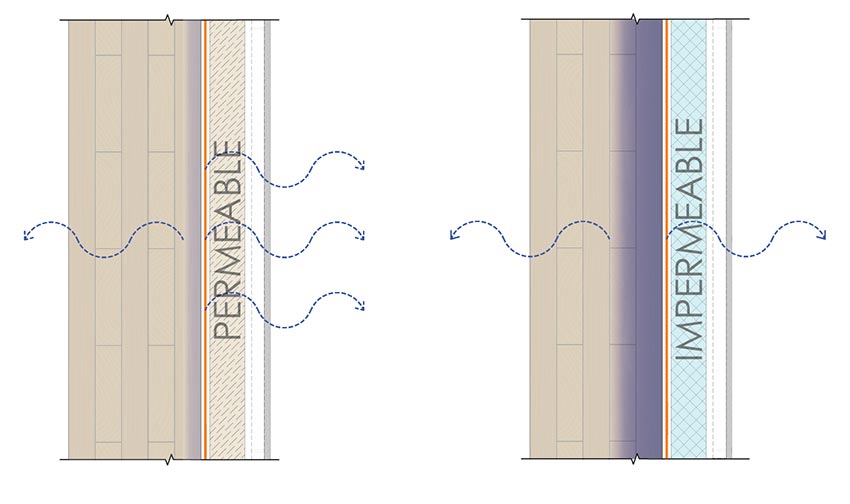
Figure 2-8
These figures show the difference between vapor-permeable exterior insulation (left) and vapor-impermeable exterior insulation (right) over a vapor-permeable membrane. Vapor-impermeable insulation can impede the drying of moisture stored in the outer CLT laminations.