How to Calculate the Wood Carbon Footprint of a Building
Learning Objectives:
- Explain what a carbon footprint is in the context of building materials.
- Describe the difference between life-cycle assessment (LCA), environmental product declaration (EPD), and whole-building LCA.
- Identify different whole-building LCA tools and how they can be used to develop a whole-building carbon footprint.
- Define what is and is not included in a wood EPD and why.
- Discuss the biogenic forest carbon cycle, and ways to track and assure forest sustainability in North America.
Credits:
This course is approved as a Structured Course
This course can be self-reported to the AANB, as per their CE Guidelines
Approved for structured learning
Approved for Core Learning
This course can be self-reported to the NLAA
Course may qualify for Learning Hours with NWTAA
Course eligible for OAA Learning Hours
This course is approved as a core course
This course can be self-reported for Learning Units to the Architectural Institute of British Columbia
From an environmental perspective, it is widely known that buildings matter. Buildings consume nearly half the energy produced in the United States, use three-quarters of the electricity, and account for nearly half of all carbon dioxide (CO2) emissions.1 The magnitude of their impacts is the driving force behind many initiatives to improve tomorrow’s structures—from energy regulations and government procurement policies, to green building rating systems and programs such as the Architecture 2030 Challenge. The focus on energy efficiency, in particular, has led to widespread improvements, so much so that many designers are now giving greater attention to the impacts of structural building materials. This greater attention has revealed that greenhouse gas (GHG) emissions associated with materials used in buildings and construction account for 28 percent of building sector emissions and 11 percent of global GHG emissions.2
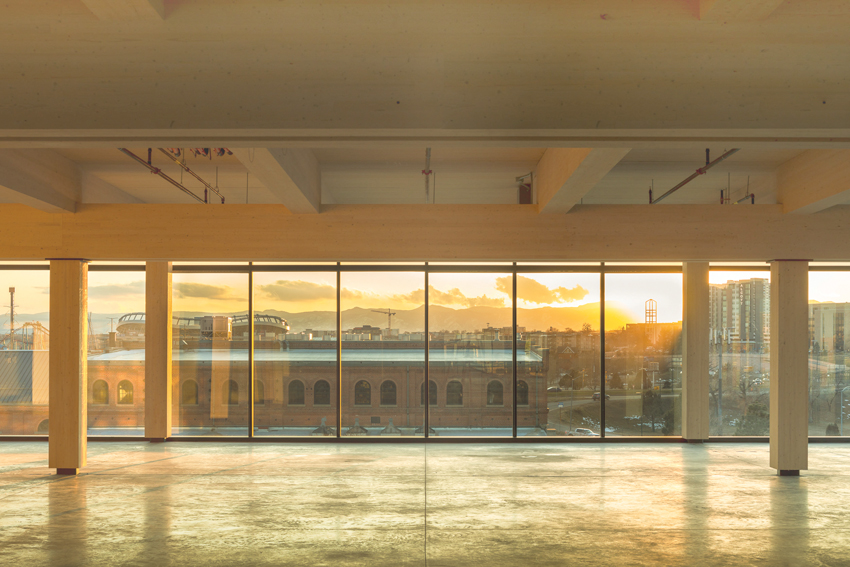
Photo: © Oz Architecture/JC Buck; courtesy of WoodWorks
Located in downtown Denver, Platte Fifteen is a five-story workspace that incorporates a mass-timber frame built using glue-laminated timber (glulam) beams and columns as well as cross-laminated timber (CLT) floor and roof panels.
Are we able to dive deeper into these numbers to find ways to reduce a building’s carbon footprint in meaningful ways? What are the methods used to measure building material carbon footprint and do they tell the whole story? Are there simple tools to assess material choices? This course seeks to address these and other questions by explaining the principal methods and tools that are used to assess carbon footprint in the context of building materials. It includes a primer on product terminology, including life-cycle assessment (LCA), environmental product declarations (EPDs), carbon footprint, embodied carbon, and whole-building LCA (WBLCA) tools. It explains how biogenic carbon is treated in standard LCA methodology and dives into the forest side of the equation, explaining basics of the sustainable forestry cycle. This course also highlights some ways to track and assure wood comes from sustainable forests in North America and why demand for wood products supports investment in forest management.
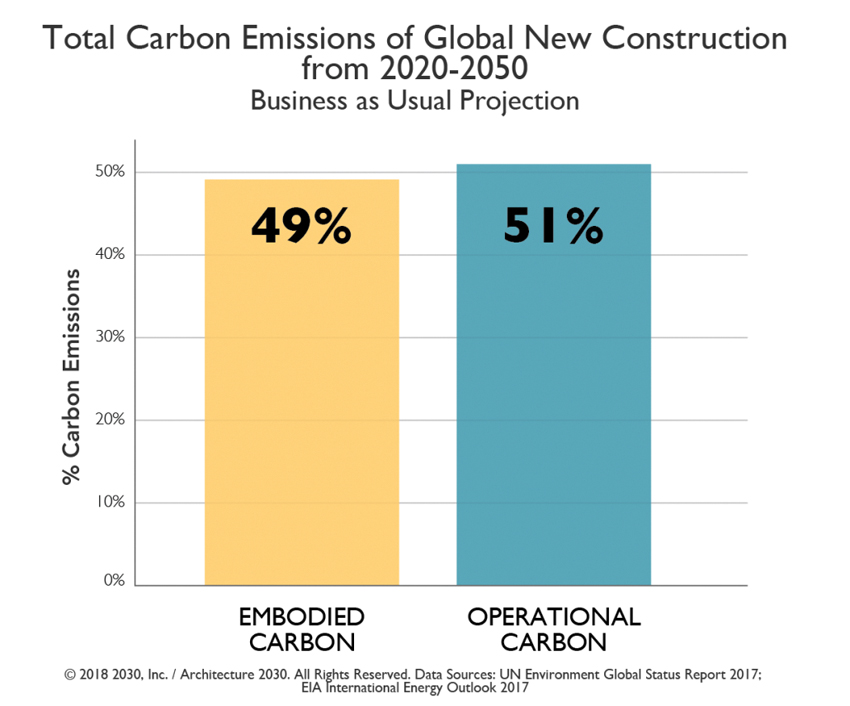
Source: © 2018 2030, Inc./Architecture 2030. All Rights Reserved. Data Sources: UN Environment Global Status Report 2017; EIA International Energy Outlook 2017. www.architecture2030.org
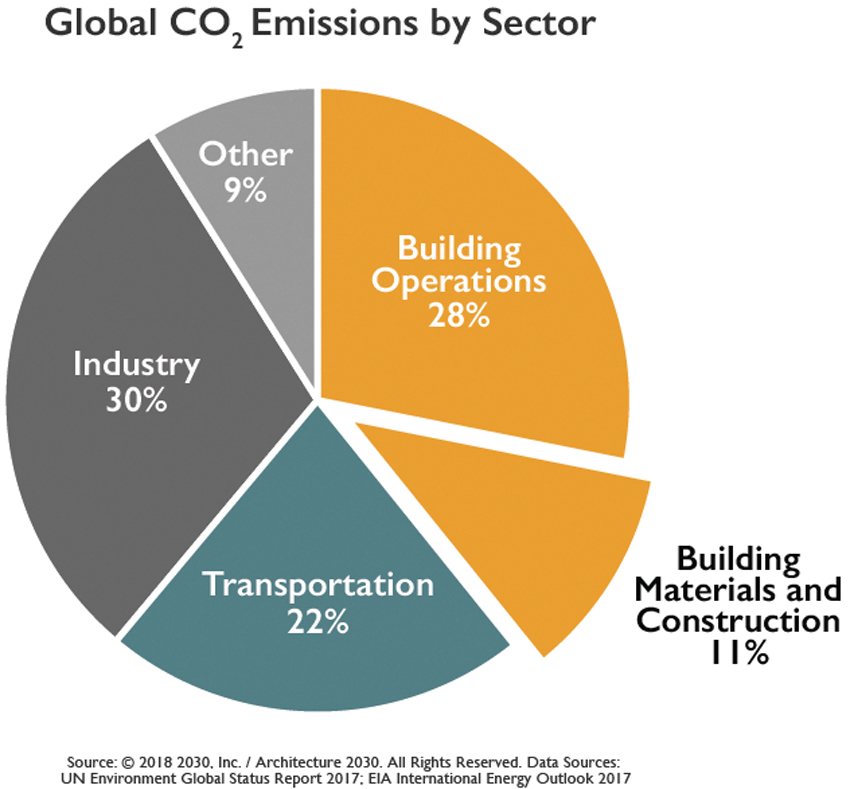
Source: © 2018 2030, Inc./Architecture 2030. All Rights Reserved. Date Sources: UN Environment Global Status Report 2017; EIA International Energy Outlook 2017. www.architecture2030.org
Why Buildings Matter
The growing urgency to address climate change was underscored in a 2018 Special IPCC report warning that in order to allow time for the earth to adapt to a warming climate, carbon emissions need to be further reduced to be in line with a 1.5 degrees Celsius warming, not 2 degrees Celsius as originally discussed. They warned that a 2 degrees Celsius temperature rise could raise sea levels to such a height that another 10 million people would be at risk of flooding. To stay within 1.5 degrees Celsius warming, global GHG emissions need to decline 45 percent below 2010 levels by 2030 and reach net-zero emissions by 2050.
The building sector has a critical role to play in what happens over the next 30 years. Not only do buildings account for almost 40 percent of global GHG emissions, but the increasing urbanization of the population means that 2.48 trillion square feet of building is expected to be added to the global building stock by 2060. This number is essentially double the current building stock, making the choice of materials in buildings over the next decades that much more important.
Architects and engineers are setting aggressive goals to help reduce emissions. Architecture 2030 has issued the following challenge to the global architecture and building community for embodied carbon: “The embodied carbon emissions from all buildings, infrastructure, and associated materials shall immediately meet a maximum global warming potential (GWP) of 40 percent below the industry average today. The GWP reduction shall be increased to: 45 percent or better in 2025; 65 percent of better in 2030; zero GWP by 2040.”3 The 2030 Challenge is widely adopted in the United States and globally by individuals, professional organizations, and governments. It has been adopted into federal, state, and local government legislation and has become standard practice in many AEC firms. It advances the use of low-carbon/carbon-sequestering materials, building materials with high-impact potential for emissions reductions, and whole-building approaches to emissions reductions.
Similarly, the structural engineering community has set the SE2050 goal to promote, design, and construct net-zero embodied carbon structures by 2050 (se2050.org). “Signing up to the SE 2050 Commitment Program requires creating a firm-wide plan to reduce the embodied carbon of your structural systems, tracking your progress, and evaluating and reducing the embodied carbon impacts of the design decisions you make on your projects.”
Carbon Footprint in the Context of Building Materials: A Primer on the Terms
Understanding a material’s impact at every stage of its life is essential for designers looking to compare alternate designs or simply make informed choices about the products they use. Life-cycle assessment (LCA) is an internationally recognized method for measuring the environmental impacts of materials, assemblies, or whole buildings from extraction or harvest of raw materials through manufacturing, transportation, installation, use, maintenance, and disposal or recycling.
An LCA is sometimes described as cryptic and complicated. Yet, what is involved is simply a thorough accounting of resource consumption, including energy, emissions, and wastes associated with production and use of a product. For a “product” as complex as a building, this means tracking and tallying inputs and outputs for all assemblies and subassemblies—every framing member, panel, fastener, finish material, coating, and so on. To ensure that results and data developed by different LCA practitioners and in different countries are consistent, LCA practitioners must adhere to a set of international guidelines set forth by the International Organization for Standardization (ISO).
An LCA assesses many different environmental impacts, but the one that many builders and architects are interested in from a climate perspective is carbon footprint. A building carbon footprint includes both operation emissions and the emissions associated with producing the material. A building material carbon footprint is often referred to as “embodied carbon.” Note that embodied carbon refers to the emissions associated with manufacturing a product, not the carbon that is physically stored in the wood product itself.
One of the reasons wood tends to have lower embodied carbon is that it requires far less energy to manufacture than other materials4—and very little fossil-fuel energy, since most of the energy used comes from converting residual bark and sawdust to electrical and thermal energy. For example, the production of steel, cement, and glass requires temperatures of up to 3,500 degrees Fahrenheit, which is achieved with large amounts of fossil-fuel energy. Member companies of the American Wood Council, on average, rely on residual bark and wood biomass for more than 75 percent of their energy needs.5