All About Glass & Metals: Guide for Architects
Learning Objectives:
- Discuss how new glass and glazing techniques can support energy-efficient building requirements and meet sustainability and carbon-reduction goals in a variety of project types.
- Identify innovations in insulating glass technologies used in the highlighted projects to provide a healthy and comfortable building environment.
- Explain the importance of thermal bridging in the façade, how metal penetrations in wall assemblies can significantly reduce the effective R-value of the wall insulation, and the role that glass and glazing products play in helping to mitigate potential issues.
- Describe the balance of daylighting and glare to optimize occupant comfort and wellness, as well as performance and cost benefits of specific glass and glazing techniques.
Credits:
This course is approved as a Structured Course
This course can be self-reported to the AANB, as per their CE Guidelines
Approved for structured learning
Approved for Core Learning
This course can be self-reported to the NLAA
Course may qualify for Learning Hours with NWTAA
Course eligible for OAA Learning Hours
This course is approved as a core course
This course can be self-reported for Learning Units to the Architectural Institute of British Columbia
The high-performance facade equation is a challenge to solve. Design and project teams must weigh a myriad of factors to develop facade systems that meet next-level energy efficiency requirements, optimize whole-building performance, provide a healthy and comfortable environment for occupants, achieve increasingly important sustainability and carbon-reduction goals, meet budget, and more.
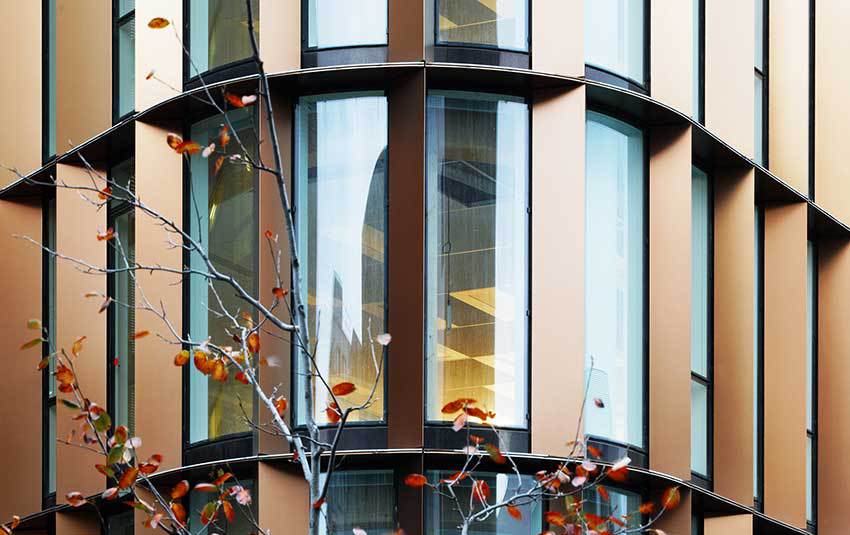
Photo courtesy of Takuji Shimmura
The BNP Curve in Paris features a more than 100,000-square-foot facade that allows the interior to be flooded with natural daylight. The insulating glass includes transparent and highly selective solar control glass from Saint-Gobain and warm-edge 16-millimeter Super Spacer Triseal Premium Black Plus from Edgetech/Quanex Building Products. The architect was Chartier Dalix Architects.
The good news for the building industry is that glass and glazing technologies exist to meet even the most stringent performance goals. “We can get to the point where the facade glazing systems can be better than the insulating opaque wall for any location, any orientation, any glass area,” according to Stephen Selkowitz, principal, Stephen Selkowitz Consultants, affiliate, Lawrence Berkeley National Laboratory, speaking during a presentation at the January NGA Glass Conference: Long Beach. However, meeting the wide-ranging goals for performance comes with mighty challenges. “This is not easy,” and it can be expensive, says Selkowitz.
The National Glass Association and Glass Magazine’s 2022 All About Glass & Metals: Guide for Architects takes a closer look at three essential elements to the high-performance facade equation. Part 1: Performance, explores the often-overlooked topic of thermal bridging in the facade; Part 2: Products, looks at innovations in insulating glass technologies; Part 3: People, considers balancing daylighting and glare to optimize occupant comfort and wellness; and Part 4: Payback, answers three key performance cost-benefit questions.
This article is part of the National Glass Association’s and Glass Magazine’s in-depth All About Glass & Metals series for architects and specifiers. The complete series also addresses considerations for protective glazing, interior glass, glass and glazing specifications, complex facades, and more.
PART 1: PERFORMANCE
THE IMPORTANCE OF THERMAL BRIDGING
“Thermal bridging is a big deal. It is the silent degrader,” said Helen Sanders, leader in strategic business development for Technoform, during the meeting of the energy group of the NGA Fabricating Committee during the NGA Glass Conference: Long Beach held in January.
Thermal bridging occurs when building exterior cladding or structural components with higher thermal conductivity penetrate or bypass insulation, thus creating a path of least resistance for heat transfer. For example, a steel beam or balcony penetrating the wall insulation will create a path for heat transfer. This pathway could lead to heat loss and decreased energy efficiency as well as potential cold points that can lead to condensation within the building envelope on exposed, interior surfaces.
“We’re not talking about thermal bridging in the frame. That is accounted for with U-factor,” described Tom Culp, owner of Birch Point Consulting, during the conference. “This [is] about how [heat transfer] bypasses insulation in a building. … It’s about alignment between the wall and the windows.”
In wall assemblies, metal penetrations can significantly reduce the effective R-value of the wall insulation. For fenestration assemblies, the potential for thermal bridges may occur where the window framing meets the surrounding wall if the surrounding insulation layer is bypassed.
Source: Adapted and excerpted from the “Thermal Bridging Considerations at Interface Conditions Design Guide,” published in January by the National Glass Association.
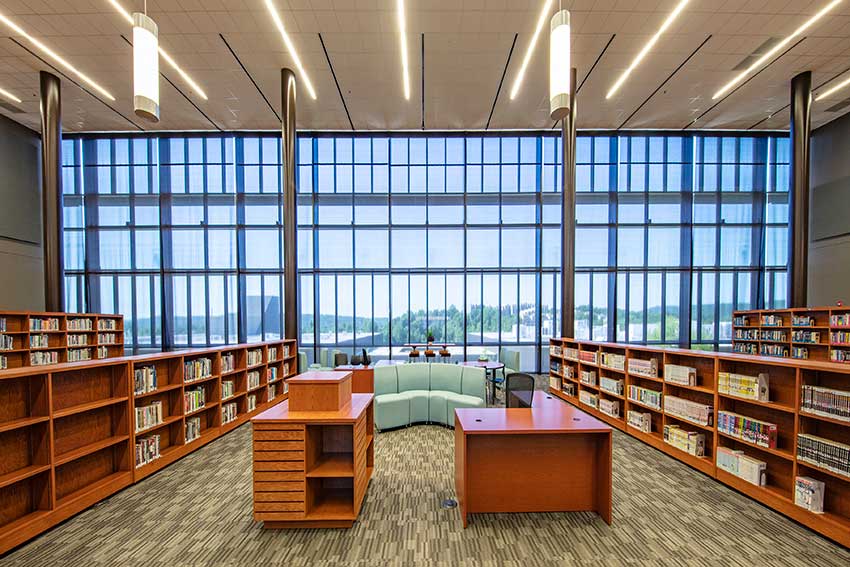
Photo courtesy of Kawneer
The interior of the new Fayetteville High School in Fayetteville, Ark., is illuminated by 28,000 square feet of curtain wall and 14,000 square feet of storefront glass, creating an open, airy design that helps foster a healthy environment for students and teachers.
Thermal Bridging in the Codes
Model energy codes typically address the performance of individual components in the building envelope. This includes the U-factor and solar heat gain coefficient of fenestration products as well as the R-value of opaque wall construction and roofing assemblies.
Model energy codes typically have not addressed the intersections of these components or penetration of the insulation; however, as they increase in overall stringency, some codes are beginning to address thermal bridging. For example, the Seattle Energy Code increases the amount of continuous insulation required in opaque wall construction based on percent area of metal penetrations compared to a wall with minimal metal penetrations (or thermal bridging). Energy codes in Vancouver and New York City require that thermal bridging details be identified on drawings and be accounted for in all performance modeling.
Most notably, ASHRAE 90.1 has been working on comprehensive new requirements to mitigate thermal bridges in many areas including roof edges, parapets, intermediate wall edges, balconies, masonry shelf angles, and the wall-to-fenestration intersection. These requirements will apply to colder regions (ASHRAE’s climate zones 4-8) and are anticipated to be included in the 2022 edition of ASHRAE 90.1, which will also be referenced in the 2024 International Energy Conservation Code (IECC).
How to Account for Thermal Bridges
The energy codes account for thermal bridges in two ways: simple prescriptive strategies and more complex modeling.
The simple approach can be deduced to a basic concept of a “thermal line” or “thermal plane.” Just as designers and engineers look for a continuous line connecting the constructions to form the primary air and water barriers, they should also now look to construct a continuous thermal line connecting the insulating materials across the transition details from fenestration to surrounding construction to create a “thermal barrier” between components. This results in different simple prescriptive strategies, shown in the diagrams pictured.
Strategies to Consider
For fenestration assemblies, whether a punched opening window or a curtain wall, the concept of a “thermal line” leads to the ideal scenario: alignment of the insulating glass units, thermal breaks within the frame, and insulation in surrounding construction without any significant bypasses. This ideal is not always possible when considering the many functional design requirements regarding structural support, water management, etc. However, better alignment will generally result in less thermal heat loss and an improved (lower) psi-factor.
The simplified prescriptive requirements proposed for ASHRAE 90.1 also try to encourage this practice of alignment and/or a continuous thermal line as much as possible. ASHRAE 90.1’s primary requirement is to align the glazing layer within 2 inches of the wall continuous insulation (e.g. mineral wool or insulating foam board), or within 2 inches of the exterior side of the cavity insulation where there is no continuous insulation. Ideally, thermal breaks within the frame are also aligned with the glazing, and the benefit from doing so will be reflected in the lower U-factor rating for the product.
Aligning the glazing system and the wall insulation is not always possible because of functional considerations such as structural design or simply for design aesthetics. Therefore, ASHRAE 90.1 also includes other options for recessed windows or offset construction. In that case, the goal is to continue the thermal line from the continuous insulation to the window framing, covering any exposed area with insulating material with R-value greater than 3 or thermal conductivity less than 3.0 Btu·in/h·ft2·°F (this would also include the option to mount the fenestration within a wood buck). It is not required that the insulation be under the frame, as careful consideration must be given to the attachment and support of the window, as well as proper water drainage.
In all cases, the intent is to maintain a continuous thermal line, as much as possible, to minimize interruption of the thermal line, thus creating “thermally bridged” details. However, there are many other issues that must also be considered at the same time.
More from the Design Guide
Download the complete design guide at glass.org/store for definitions of the two thermal bridging characterizations, linear and point; a breakdown of complex thermal bridging modeling; diagrams and photography depicting thermal bridging concepts; and strategies for edges and perimeters. The guide also explores the responsibility of designers to indicate thermal lines in construction drawings, and the responsibility of fenestration industry companies to provide an overall energy rating for a glazing system.
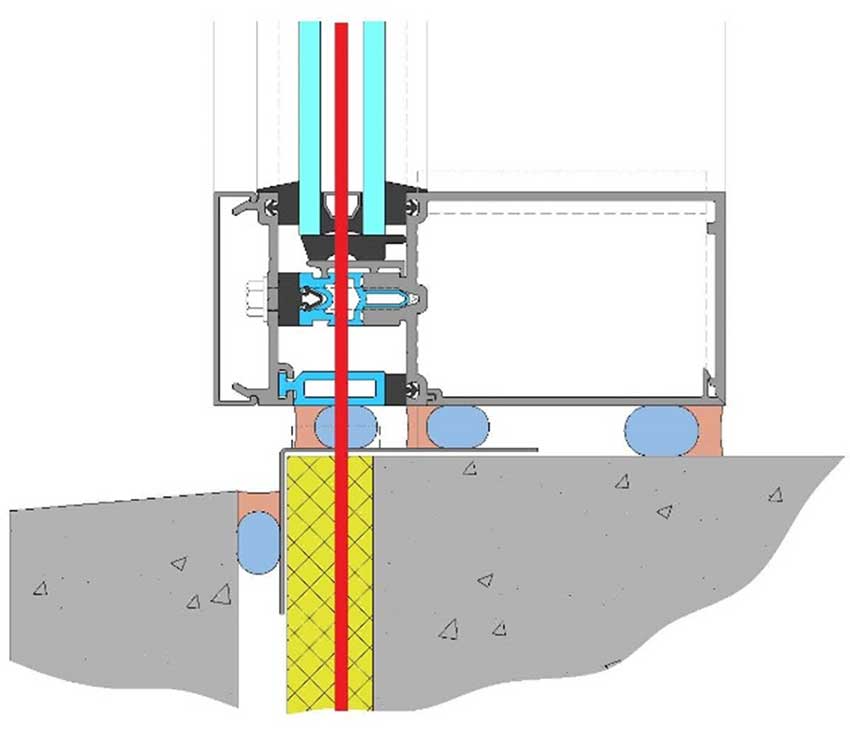
Image courtesy of National Glass Association
Efficient: Well-aligned glazing without conductive bypasses (thermal line illustrated in red).
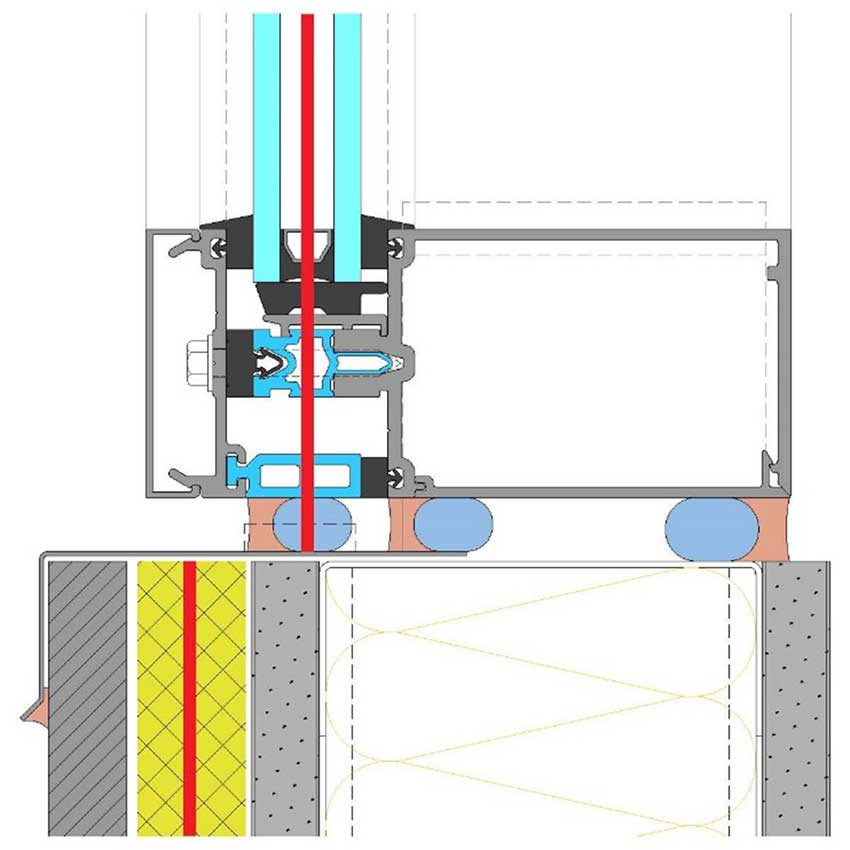
Image courtesy of National Glass Association
Regular: Misaligned glazing and minor conductive bypasses (thermal line illustrated in red).
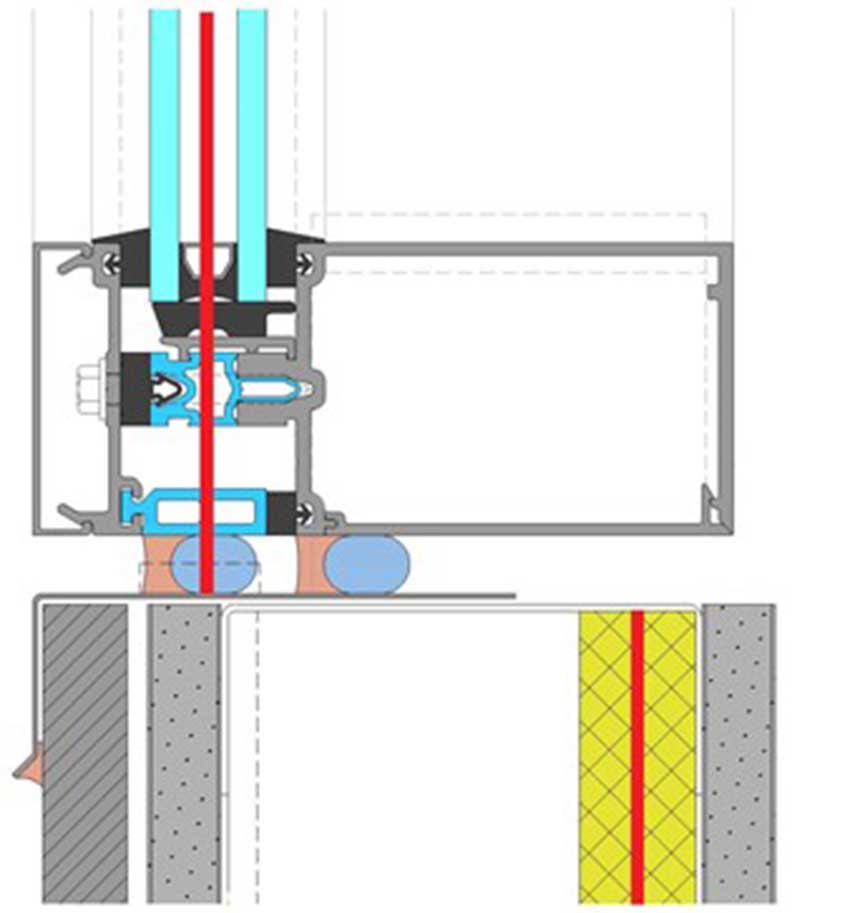
Image courtesy of National Glass Association
Poor: Cavity-insulated and conductive bypasses (thermal line illustrated in red)
The energy codes account for thermal bridges in two ways: simple prescriptive strategies and more complex modeling.