In Pursuit of Acoustical Equity
Spectral
The spectral component of sound is a more nuanced topic. Just as visible light is comprised of a range of wavelengths, sound, as we hear it, is the result of combinations of frequencies.
Singular—or discrete—frequency values are called tones, and we are able to hear between approximately 20 and 20,000 hertz (Hz). To simplify reporting data for the nearly 19,980 individual frequencies, it is common practice to divide this range into sections called “fractional octave bands.” The customary fractions are full octave bands (also referred to as 1/1) and one-third octave bands (or 1/3). Between 20 and 20,000 Hz, there are 29 one-third octave bands. The combination of all audible frequencies of a sound sum to its overall level.
It is possible for two sounds equal in overall level to be perceptibly different. Borrowing descriptors from the American Society of Heating, Refrigerating and Air-Conditioning Engineers, one can generally state that a sound that has too much low-frequency content is too rumbly, while a sound that has too much high-frequency content is too hissy, and sound that has too much mid-frequency content has a strong hum or buzzing quality.
If empowered with the ability to adjust the frequency content for a fixed level of sound (e.g., 45 dBA), there exists a favorable combination of frequencies that is most comfortable or balanced. This shape of sound is documented in literature by Beranek (and BBN) and Warnock—and, more recently and precisely, by the National Research Council (NRC)—and it forms the basis for the synthesis of masking sound.14 When professionally tuned to meet this shape (typically called a spectrum or curve) for the majority of the audible frequency range (100 to 10,000 Hz), background sound resides in the ‘Goldilocks Zone.’ Occupants’ perception of the final product may be described as quiet—free from rumble, hiss, or buzz and absent of hum or buzzing. Furthermore, the overall level is neither too high to disturb occupant comfort, nor too low to compromise acoustical privacy.
Spatial
The spatial component of sound is no less complex. It refers to the variability of the level—also, inherently, that of the spectra—of sound, in space. These variations are a function of many parameters, including not only the source and location from where the sound originates (e.g., building systems, occupants, appliances, and even oneself), but also the space’s architecture (i.e., size, shape, geometry) and fit out (i.e., finishings, fixtures, furnishings).
As sound from a source is generated, it propagates with its level decaying as a function of distance, and by the number of times it is reflected (loses energy) from other surfaces or at room boundaries. While its energy continually dissipates, its eventual inaudibility is not because its level is attenuated below one’s auditory threshold but because it drops below the background sound in one’s environment—the background sound that actually exists. This phenomenon is known as the Masking Effect, where the background sound covers the propagating noise. Figures 3 and 4 provide simplified modeling of this effect. Not only does masking sound reduce the distance over which a noise can be heard (sometimes referred to as the radius of distraction), but it also creates a more consistent—and equitable—acoustical experience for occupants, both in their individual work areas and as they move throughout the space.
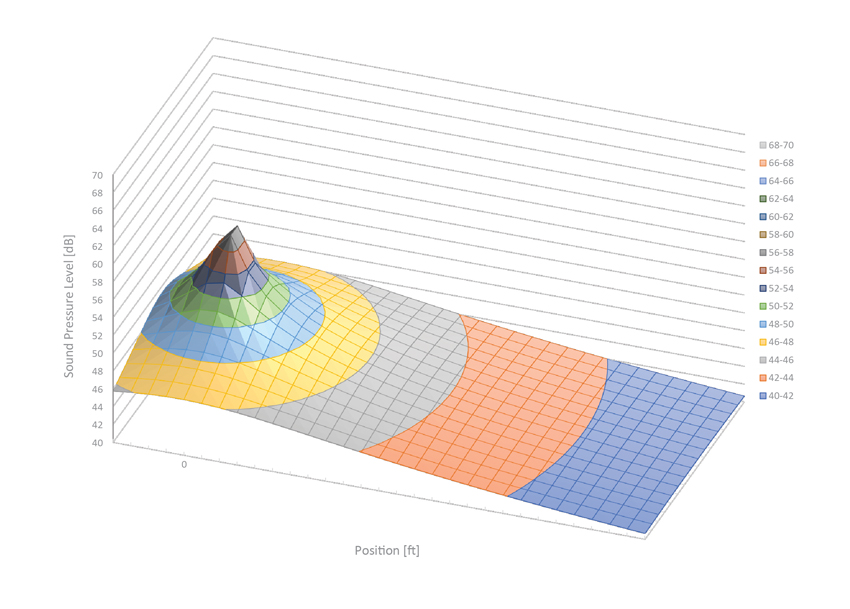
Images courtesy of K.R. Moeller Associates Ltd.
Figure 3: This simplified model shows how sound propagates as it moves away from a source, across—or rather, throughout—a space. Each notch along the horizontal axis represents 1 foot, with 0 marking the origin of the noise.
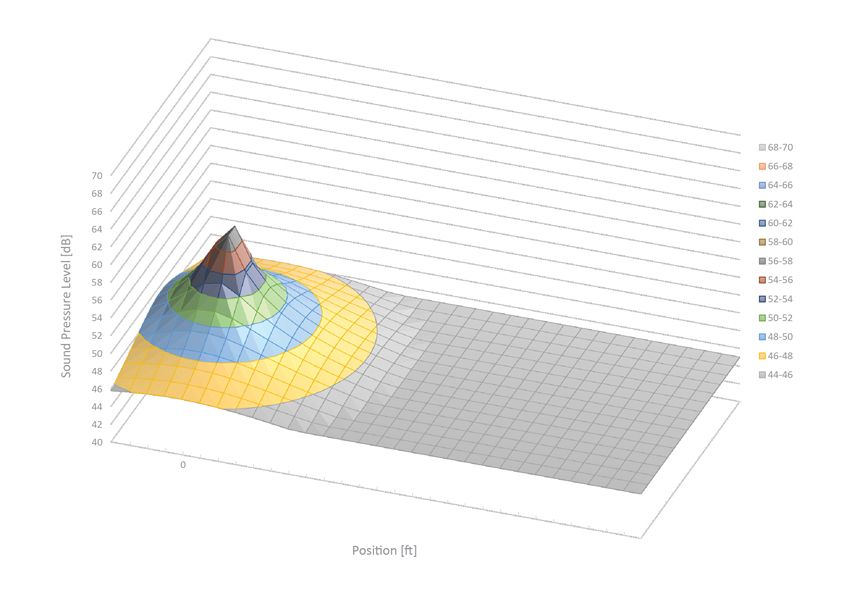
Figure 4: This simplified model shows how masking reduces the distance over which the noise shown in Figure 3 can be heard. The effect is noticeable in terms of where the propagating signal reaches and falls below the level of masking sound (grey shaded area).
Control versus Cover
While many still associate the C in the ABC Rule with cover, control is a more accurate term, for a number of reasons.
Use of the word cover can unintentionally reinforce the view that this crucial element of architectural acoustics simply involves placing any sound overtop of others—like a blanket—strengthening the historical misperception that only level matters; in other words, that a sound only needs to be louder than other sounds to provide the masking effect and hence meet the requirements of C. This misperception opens the door to commoditization of sound-masking systems—the notion that the effect will simply be provided by the product, rather than in tandem with a service that ensures the sound actually meets the specified masking spectrum.
The study of architectural acoustics demonstrates that the physics of the behavior of sound within the built environment is exceedingly complex—and this is true for any sound, even that introduced via a sound-masking system. Regardless of the sophistication of the technology, the system’s layout or loudspeaker orientation (e.g., upward-facing within the plenum or downward-facing using cut-throughs), the masking effect can only be achieved through skilled field commissioning—or tuning—which adapts the sound actually produced in the room/space by accounting for its architecture and fit out. Small zones (i.e., no larger than one to three loudspeakers in size) offering fine volume (in 0.5 dBA steps) and frequency (1/3-octave) adjustment capabilities provide the technician with frequent and precise control points across the environment, helping to consistently achieve the masking effect throughout the space and hence a better outcome for the occupants.
Post-installation tuning and performance verification are both crucial to ensuring that the sound-masking system is in fact effectively controlling the spectrum and level of the sound that actually exists within the built environment—and hence dependably providing the masking effect throughout the space. It is only under these assured conditions—temporally, spectrally, and spatially consistent acoustics—that occupants can appreciate acoustical privacy.
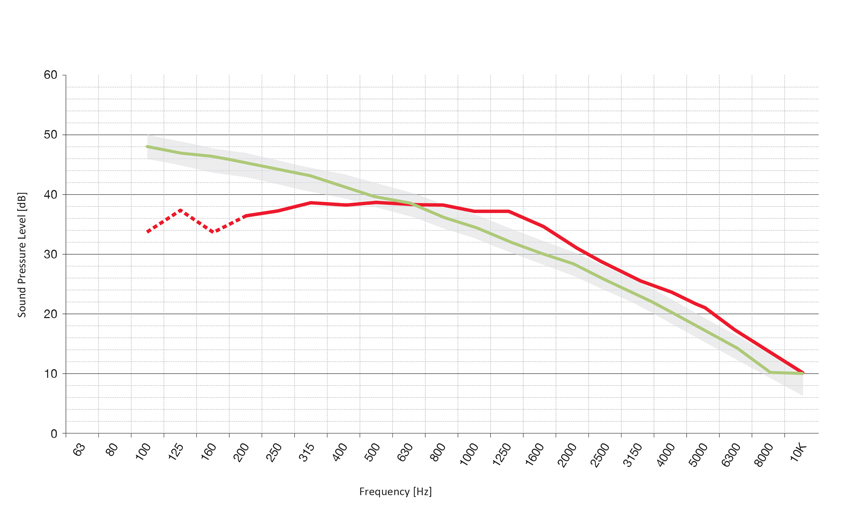
Images courtesy of K.R. Moeller Associates Ltd.
The behavior of sound within the built environment is highly complex, including that introduced via a sound-masking system, regardless of its design or the orientation of its loudspeakers. If the measured output—the background sound actually produced in the space—is to meet the specified spectrum, the system must be professionally tuned post-installation. Here, a tuned system (green line) with upward-facing, in-plenum loudspeakers meets the NRC spectrum (grey shaded area), while an untuned system (red line) featuring download-facing or direct field loudspeakers fails to do so. Also note that, in the latter case, levels below 200 Hz (dashed red line) are contributed by building systems rather than the loudspeaker.
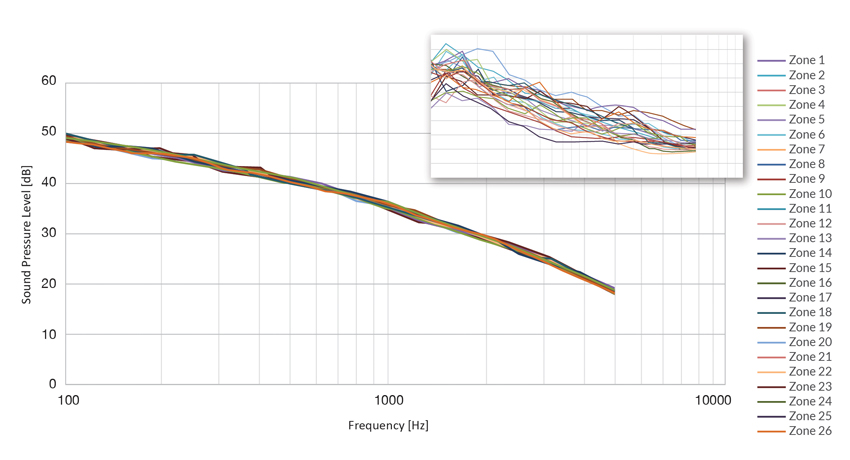
These background sound-level measurements were taken in 26 locations within an open plan. Without masking (inset), the occupants experienced varying acoustical conditions across the space. Since masking sound was applied and tuned to reliably meet the NRC masking spectrum within each small zone, occupants experience a far more consistent level of acoustical privacy and comfort throughout the space.