Reducing Embodied Carbon in Concrete
Learning Objectives:
- Explore the evolution of concrete and supplementary cementitious materials (SCMs) as they relate to safely designed buildings.
- Investigate the environmental issues related to using concrete as a primary building material, particularly in terms of embodied carbon.
- Assess Portland-limestone cement (PLC) as an alternative blended cement with reduced embodied carbon that helps create more sustainable buildings.
- Specify Type IL Portland-limestone cement into project specifications consistent with approved standards for safety and performance.
Credits:
This course is approved as a Structured Course
This course can be self-reported to the AANB, as per their CE Guidelines
Approved for structured learning
Approved for Core Learning
This course can be self-reported to the NLAA
Course may qualify for Learning Hours with NWTAA
Course eligible for OAA Learning Hours
This course is approved as a core course
This course can be self-reported for Learning Units to the Architectural Institute of British Columbia
Concrete has been used widely in buildings for centuries. It is a remarkable material. When newly mixed, concrete is plastic and malleable. When cured and hardened, it is strong and durable. Its ubiquitous presence attests to its strength, versatility, durability, and utility as a primary construction material. However, the production and creation of concrete, primarily the cement used to make it, emits large amounts of CO2 into the atmosphere. This course looks at the use of Portland-limestone cement (PLC) as a proven alternative to ordinary Portland cement (OPC) in concrete. The addition of supplementary cementitious materials (SCMs) such as fly ash, slag, and natural pozzolans is also addressed. These strategies have been recognized as a way to significantly reduce the amount of CO2 embodied in the concrete.
The Evolution Of Concrete As A Building Material
In order to understand the nature of concrete and its significance in construction, it is important to understand both concrete's make up and history.Fundamentally, concrete is a mixture of aggregates such as naturally derived stone, sand, or other materials that are held together using a hydrated paste made from cement and water. Cement is created from processed natural materials that react chemically with water in a process known as hydration. This chemical reaction with water transforms the fluid paste mixture (i.e., cement and water) into a solid material which develops strength over time. It also firmly binds the aggregates together into the rock-like mass we have come to know as concrete.
The specific materials used to make concrete and cement have varied over time and have informed our modern understanding of how to make better concrete today.
Pre-Roman Concrete
The earliest known use of rudimentary concrete dates back to about 6,500 B.C. in the Middle East—current-day southern Syria and northern Jordan. Nabataean Bedouins who controlled oases in this desert area were interested in creating places to store water. They found they could mix some local deposits of silica sand, limestone, and pozzolan together to create a rather waterproof enclosure. Pozzolan is a common sandy volcanic ash that exists in many areas around the world in different types and with different properties. These Bedouins used a very dry mix of materials with only a little water which they would tamp into place by hand, making it more gel-like with greater bonding.
The ancient Egyptians around 3,000 B.C. used lime-based mortars to hold stone and bricks together in the pyramids and other structures. By 600 B.C. the ancient Greeks discovered a natural pozzolan that formed cementitious compounds, called calcium silicate, when mixed with lime and water. Although they used it somewhat selectively for buildings, its properties were strong and durable. This mixture is found in structures that are still standing today.
Roman Concrete
The Romans were very prolific in their use of concrete and other cementitious products such as mortar (to hold large stones together) between 200 B.C. and 500 A.D. Their mix of materials typically used a drier, less plastic version than the Greeks which produced considerable strength and durability. For larger and grander structures, the Romans incorporated volcanic sand (a form of pozzolan) to react chemically with lime and water, causing cementitious hydration. This likely represented the first large-scale use of a truly hydraulic cementitious binding agent in a concrete mix and was a part of many Roman utilitarian structures like aqueducts, bridges, etc. It was also used for significant buildings, many of which are still standing today, such as some Roman baths, the Pantheon in Rome, and the Colosseum. The Pantheon is an example of cast-in-place concreting techniques, with the entire dome, made with pumice as a lightweight aggregate to lighten the weight of the structure, placed without a cold joint. The Romans also appear to be the first to experiment with what we term admixtures to enhance performance. The Roman versions included things such as animal fat, milk, and blood to adjust the physical properties of the concrete mixtures. Another sign of sophistication for the time shows up in the apparent ability of the Romans to manufacture two types of artificial pozzolan when natural pozzolan aggregate was not readily available.
Concrete Advances in the 19th Century
The Roman methods of using concrete and mortar were apparently so well developed that very little changed about its mixing and use until the early 1800s. In 1793, John Smeaton discovered a modern method producing hydraulic lime for cement by using limestone containing clay that was fired at high temperatures in a kiln, creating di-calcium silicate. The resulting stone-like product called “clinker” was then ground into powder. Soon after, in 1824, an Englishman named Joseph Aspdin took this a step further by using optimized proportions of finely ground chalk and clay in a high-temperature kiln, further improving strength performance. This created another version of clinker containing tricalcium silicate. He named this product “Portland cement” because it resembled the high-quality building stones found in Portland, England. This kiln-based method of producing cement meant a steady and consistent supply could be shipped to construction locations and mixed with other local ingredients to form concrete, an obvious benefit at the dawn of the industrial age.
Between 1835 and 1850, systematic tests to determine the compressive and tensile strength of cement were first performed, along with the first accurate chemical analyses. By 1860, Portland cements of modern composition were produced and manufactured to detailed standards important to the hydration process and the chemical characteristics of the hydraulic cement. These standards were based on heating a mixture of limestone and clay in a kiln to temperatures between 1,300 degrees Fahrenheit and 1,500 degrees Fahrenheit. In 1885, a horizontal, slightly tilted kiln that could rotate the cement ingredients and function more efficiently was developed. This rotary kiln provided better temperature control and did a better job of mixing materials, so much so that by 1890, rotary kilns dominated the market.
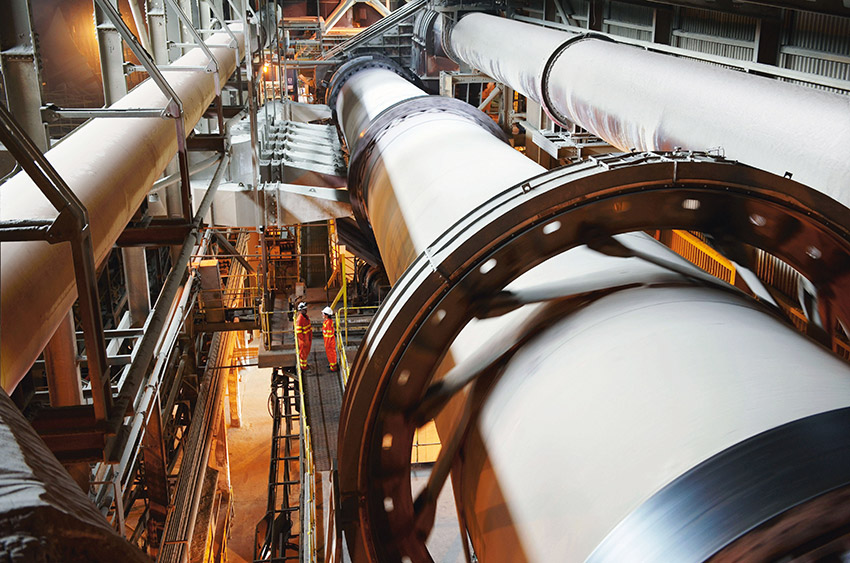
Photo courtesy of Holcim
Horizontal rotary kilns heat limestone mixed with clay or shale to temperatures between 1,300 degrees Fahrenheit and 1,500 degrees Fahrenheit to create the clinker for Portland cement.
These advancements are all significant, however, kilns operating at those high temperatures require a lot of energy. During the age of the Industrial Revolution in the 1800s and early 1900s, that meant using fossil fuels (coal, oil, or natural gas) since it was relatively cheap and available. Little attention was given to the environmental impacts of using those fuels or the recognition that each clinker produced accounted for a significant amount of carbon dioxide being emitted into the atmosphere.
In all cases, it is worth noting that CO2 is released at two points during cement production. Roughly 40 percent of the CO2 generated is from the burning of fossil fuels in the manufacturing process. Manufacturers have begun to address this by using alternative fuels such as waste fuels or renewable energy where possible which produces lower emissions. The remaining 60 percent of CO2 is from naturally occurring chemical reactions to change limestone or CaCO3 into CaO and CO2. Changing the makeup of the cement helps lower this as does the capture or sequestering of some of this CO2.