Concrete Innovations
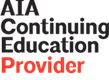
Learning Objectives:
- Describe new technologies used in concrete manufacturing that utilize recycling of materials.
- List ways in which these innovative concrete products can improve project performance.
- Explain how to implement the latest concrete innovations in building and infrastructure projects.
- Discuss the importance of incorporating new technologies to enhance resilience and sustainability in the built environment.
This course is part of the Concrete Academy
Starting in 2017, more research was done on how biochar affects the microstructure of mortar, water permeability, mechanical strength, and carbon sequestration. In 2018, research was extended to gain a better understanding of how biochar contributes to mortar/concrete, including biochar’s pore size and shrinkage, and looking at “reservoir” effect, which involves using pre-soaked biochar.
Biochar Concrete Composition
The composition of biochar concrete typically includes portland cement, aggregates, water, and biochar. Portland cement serves as the binding agent that holds the concrete together, while the coarse and fine particles of aggregates—such as sand, gravel, crushed stone, recycled concrete—make up the bulk of the concrete. Water is used to mix the cement and aggregates together, forming a paste that hardens and binds the components. Biochar is added to the concrete mix to achieve all these additional benefits such as improved strength, reduced weight, and increased resistance to moisture and temperature changes. Biochar can also enhance the concrete's ability to absorb and retain water, which can be beneficial for plant growth in green infrastructure applications. The proportions of these components may vary depending on the specific application and desired properties of the biochar concrete.
The Future or Biochar
Accelerated carbonation curing is a process in which carbon dioxide is introduced to a material to accelerate its curing or hardening. Experimentation with accelerated carbonation curing techniques for cement dates back to the early 20th century, and in the decades that followed, it became a widely used technique. In the 1960s and 1970s, research focused on optimizing the process to improve the strength and durability of concrete. Researchers developed a range of techniques for controlling the curing environment, including using curing chambers, greenhouses, and carbon dioxide injection systems.
One of the key benefits of carbonation curing is its ability to significantly reduce the time required for concrete to reach its full strength. This has made it a popular technique in construction projects where time is a critical factor. For example, carbonation curing has been used in the construction of high-rise buildings, bridges, and other large-scale infrastructure projects.
More recently, researchers have focused on the potential environmental benefits of carbonation curing. The process can help to reduce the carbon footprint of concrete production by sequestering 2 in the material. Some researchers have even proposed using carbonation curing as a means of carbon capture and storage, where CO2 from industrial processes is captured and injected into cement-based materials for permanent storage.
Using biochar is yet another strategy for accelerated carbonation curing. When biochar is added to the mix, it provides a large surface area for carbon dioxide to react with. The reaction leads to the formation of calcium carbonate, which is the same material that is formed during the natural carbonation of concrete. As discussed earlier, adding biochar to concrete sequesters carbon and increases porosity, reduces cracking, and improves its durability. It is still on the cutting edge: To date there are only about 15 articles about biochar for accelerated carbonation curing and the research lacks a unified framework to compare one set of results over a long period of time.
To make it more commercially viable, a material standard needs to be developed to ensure uniformity in biochar quality and safety of concrete components, such as providing acceptable levels of heavy metals and toxic substances. There will also need to be more work put into biochar applications and products that compete with current products, which might entail developing cheaper biochar blends. And, in general, biochar needs more test-bedding projects to play out rigorous, transparent, and replicable testing of biochar theories. It is a young technology, but offers a promising area of research that has the potential to improve the sustainability and efficiency of concrete production.
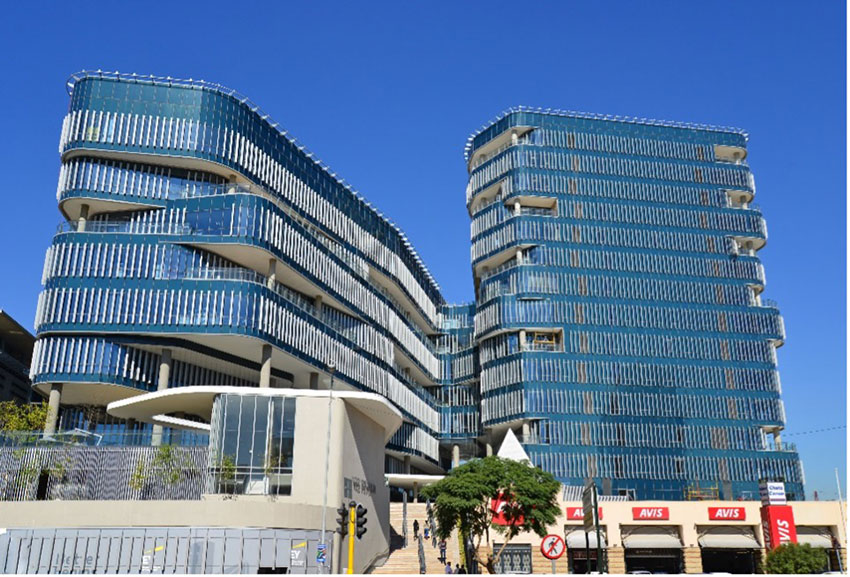
Photo courtesy of Greg Balfour Evans/Alamy Stock
102 Rivonia in Johannesburg, South Africa, used fly ash in the concrete to reduce the overall cement use of the project by 30 percent, which heavily contributed to the project having a lower carbon footprint.
INNOVATIVE WAYS TO EXPAND THE SUPPLY OF FLY ASH
Fly ash is the most plentiful of all SCMs and is roughly half the cost of portland cement. However, because of increased emissions regulations on coal-fired power plants, not nearly as much high-quality fly ash is produced as in the past. In addition, with a move towards renewables and natural gas, coal-fired power plants are closing with many cost-effective supplies diminishing.
While coal power generation started in the early 1900s in the U.S., the use of fly ash in concrete only started to reach significant volume in the late 1900s, and it is estimated that about 1.5 billion tons of coal ash has been placed in landfills, of which some is fly ash. This is where innovation comes in. Several companies, understanding a likely increased demand for fly ash in concrete, began to recover fly ash from landfills and treat it using a process called beneficiation.
Beneficiation simply means taking coal ash from landfills and processing it to meet the necessary standards for beneficial use. For fly ash, that typically means reducing the amount of unburned carbon in the ash. Carbon tends to have an absorptive quality which inhibits air-entraining and water-reducing admixtures. There are also other chemicals such as ammonia in some coal ash deposits which must be reduced before use in concrete.
Several companies have developed processes for harvesting ash from landfills and reducing the unburned carbon and ammonia, calcium, sulfur, and other impurities. The simplest process is to burn off the excess carbon. Still other methods use chemical treatment to mitigate the effects of carbon and ammonia, and one company uses low-frequency sound to reduce particle size and make it more uniform, a desired characteristic of fly ash.
According to the article “Digging Through the Past: Harvesting Legacy Ash Deposits to Meet Future Demand,” authored by Rafik Minkara and published in Issue 1 2019 of Ash at Work magazine, Minkara concludes: “While the variety of technologies now exist to beneficiate land-filled and ponded ash, the cost and complexity of doing so can be challenging.” He goes on to say, “Beneficiation processes can be as simple as using off-the-shelf equipment or as involved as developing customized solutions with high capex requirements.” In the end, it will depend on demand for fly ash. As low-cost supplies diminish over time, the demand is likely to be filled by harvesting and beneficiating the vast supply of coal ash in landfills.
CARBON UPTAKE IN CONCRETE
Like most manmade materials, concrete is considered a CO2 emitter, mainly due to the cement manufacturing process. But what if you could reverse that process and capture or sequester CO2 in concrete through natural processes or carbon capture technologies?
Carbonation is a naturally occurring process by which CO2 penetrates the surface of hardened concrete and chemically reacts with cement hydration products to form carbonates. For in-service concrete, carbonation is a slow process with many dependent variables. The rate decreases over time. This is because carbonation decreases permeability and carbonation occurs from the surface inward, creating a tighter matrix at the surface making it more difficult for CO2 to diffuse further into the concrete. While slow, the carbonation process does result in an uptake of some of the CO2 emitted from cement manufacturing, a chemical process called calcination.
Theoretically, given enough time and ideal conditions, all of the CO2 emitted from calcination could be sequestered via carbonation. However, real world conditions are usually far from ideal.
The rate of CO2 uptake depends on exposure to air, surface orientation, surface-to-volume ratio, binder constituents, surface treatment, porosity, strength, humidity, temperature, and ambient CO2 concentration. Predicting how much CO2 is absorbed by in situ concrete is difficult. What is known is that rates of CO2 uptake are greatest when the surface-to-volume ratio is high, such as when concrete has been crushed and exposed to air.
In one of the most comprehensive studies, Xi, et al, published a summary of their research in the article “Substantial Global Carbon Uptake by Cement Carbonation,” in the journal Nature Geoscience in November 2016. The research quantifies the natural reversal of the calcination process—carbonation. Using analytical modeling of carbonation chemistry, they were able to estimate the regional and global CO2 uptake between 1930 and 2013. They estimate that the cumulative amount of 2 sequestered in concrete is 4.5 Gt in that period. This offsets 43% of the CO2 emissions from production of cement caused by the calcination process. They conclude that carbonation of cement products represents a substantial carbon sink.
Two areas of research and commercialization offer considerable enhancements to this CO2 uptake process. The most basic approach is enhanced carbonation at end-of-life and second-life conditions of concrete. This might not be considered innovative, since it would simply mean changing the way demolished concrete is collected and treated before re-use. If conditions are right, and particle size is small, crushed concrete can potentially absorb significant amounts of CO2 over a small period, such as one year or two, thus leaving crushed concrete exposed to air before re-use would be beneficial.
Other commercially viable technologies accelerate carbonation. This is accomplished either by injecting CO2 into concrete, curing concrete in CO2, or creating artificial limestone aggregates using CO2.
One company uses CO2 captured from industrial emissions, which is then purified, liquefied, and delivered to partner concrete plants in pressurized tanks. It is then injected into the concrete while the concrete is being mixed, which converts the CO2 into a solid-state mineral within the concrete. The minerals formed enhance compressive strength.
The process reduces CO2 emissions in two ways: through direct sequestration of CO2 injected into the concrete mixture and by reducing cement demand since it requires less cement to produce concrete at a specified strength.
The economic viability of this type of concrete also makes it a particularly attractive innovation. The cost of the equipment and licensing is offset by the reduction in cement. The company has installed its technology in over 650 systems operating at plants around the world, which have in turn supplied over 8 million cubic yards of concrete. This product is sufficiently available to be used now and has already been used significantly in numerous projects.
CONCLUSION
As the global population grows, the demand for concrete is only going to increase. Innovations in the concrete industry can help meet the growing demand for concrete, reduce its environmental impact, improve its performance, and expand its range of applications.
In this course we discussed some of the latest innovations in cement, such as utilizing ground-glass pozzolans, natural pozzolans, and calcined clay. We have described how biochar can effectively enhance cement while also sequestering carbon and explained how harvesting “legacy” fly ash can be one part of the solution. As standards and needs evolve, innovation is always key to staying ahead of the curve. Concrete innovation is part of the conversation when it comes to sustainable practices, and in cases like Remy Wines and DEEP, lead to cutting edge projects that serve as models for change.
Erika Fredrickson, is a writer/editor focusing on technology, environment, and history. She frequently contributes to continuing education courses and publications through Confluence Communications.