Concrete Innovations
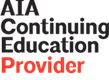
Learning Objectives:
- Describe new technologies used in concrete manufacturing that utilize recycling of materials.
- List ways in which these innovative concrete products can improve project performance.
- Explain how to implement the latest concrete innovations in building and infrastructure projects.
- Discuss the importance of incorporating new technologies to enhance resilience and sustainability in the built environment.
This course is part of the Concrete Academy
Natural Pozzolans
The use of natural pozzolans in construction dates to ancient times. The Romans used volcanic ash from Pozzuoli, Italy, to create hydraulic cement that was used in some of their most famous structures. The fact that some of those structures—like the Pantheon and the Colosseum—still exist 2,000 years later, is a testament to the material. The Pantheon, for instance, is still the largest unreinforced concrete dome on earth. The Aqueducts of Ancient Rome have been standing for even longer, surviving earthquakes. Roman concrete was made up of one part lime, three parts pozzolana, and aggregates.
The use of natural pozzolans continued throughout the Middle Ages and into the Renaissance. During the 18th and 19th centuries, the use of natural pozzolans declined as the demand for portland cement increased. But in the 20th century, interest in pozzolanic materials was renewed as researchers and engineers sought ways to improve the performance of concrete.
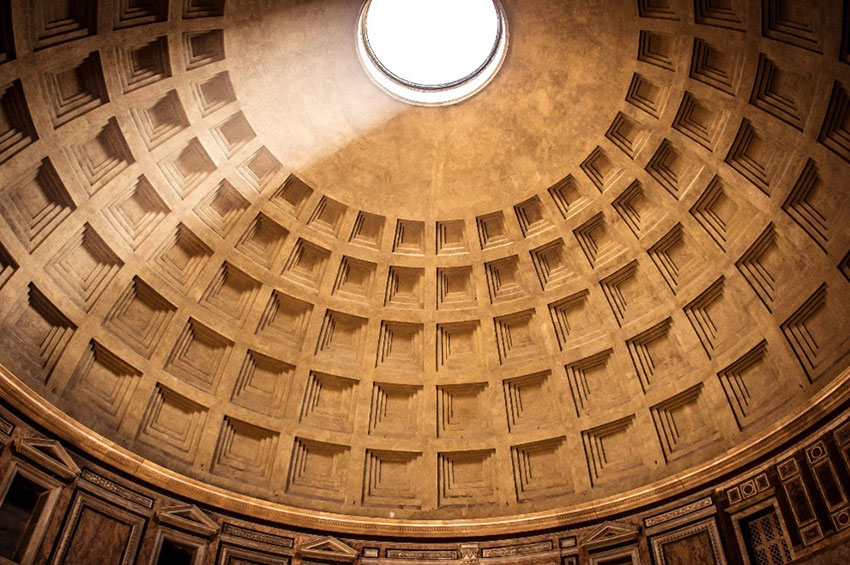
Photo courtesy of Kokhan O/Adobe Stock
The Romans built the Pantheon—which is still the largest unreinforced concrete dome on earth—with hydraulic cement made from natural pozzolans.
The first modern renaissance for natural pozzolans took place between 1900 and the 1970s when natural pozzolans were used for projects such as the Hoover Dam and Glen Canyon Dam. In fact, the Army Corps of Engineers have many projects launched during that time period throughout the West—and most of them are still standing today. In the 1970s, the concrete industry started using fly ash—a byproduct of power plants—which took the place of natural pozzolans because it was readily available and essentially free. However, as noted earlier, economic and environmental pressures have led to the shutting down of power plants. That is what has led to another renaissance for natural pozzolans, which are now being used in concrete for roads, infrastructure, wind turbine foundations, oil well cementing, homes, sidewalks, and other applications.
Characteristics of Natural Pozzolans
There are two classifications of natural pozzolan. The first are made from ejected volcanic materials such as ash, pumicite, or from diatomaceous earth consisting of the fossilized remains of diatoms, a type of hard-shelled microalgae. They have a natural silica content and can improve workability, strength, and durability of concrete or help reduce water content of cement mixes.
The second is calcined clay, a type of clay that has been heated to high temperatures to bring it back to its raw state. The high temperatures drive off organic matter and increase its pozzolanic properties. Calcined clay can be used as a natural pozzolan to improve the strength and durability of concrete mixes.
Natural pozzolans help reduce the carbon footprint of concrete products in a few ways. Raw pozzolan typically doesn’t require any heating and calcined clays are heated to less than 50% of the heat required to produce portland cement, and so they require less energy in production. Unlike limestone when it is heated for portland cement, natural pozzolans do not emit carbon dioxide during the chemical conversion process. When it is all calculated, there is an almost pound for pound reduction of carbon dioxide with raw natural pozzolans (90-93%) and 65-70% with calcined clays.
In terms of how they affect concrete, natural pozzolans can reduce permeability when used at about 25% replacement in cement. They also reduce alkali-silica reaction (ASR) and sulfate attack, which can otherwise lead to expansion, cracking, strength loss, and disintegration of the concrete. Natural pozzolans can significantly reduce or nearly eliminate efflorescence—the migration of salt to the surface—when used between 20-25% replacement rate. By incorporating natural pozzolans into concrete, you can reduce heat of hydration, which is especially important in mass concrete projects and one of the reasons natural pozzolans were used for dams in the 1970s. Finally, natural pozzolans can indirectly contribute to the curing of concrete by enhancing the strength development and improving the microstructure of the material.
Natural pozzolans are not a drop-in replacement for fly ash. They have a higher water demand than fly ash. Natural pozzolan does not heighten workability for cement, and, in fact, in can sometimes reduce it, which is why the mix has to be adjusted, usually with more water or water reducer. However, in the case of natural pozzolans—unlike some other SCMs—more water (to a point) can lead to concrete with greater compressive strength and surface resistance.
The Future of Natural Pozzolans
Currently, cement standards are based on mixes that require water reduction. New tests show that there is a window in which increased water w/cm provides optimal concrete performance and even reach higher surface resistivity levels than fly ash mixes. The prescriptive specification for water to cementitious limits does not predict the strength, permeability or resistivity with accuracy for natural pozzolans since these limits were not developed for natural pozzolan materials. The future of natural pozzolans will likely include more testing and the development of more applicable standards.
There are also a lot of questions that need to be explored about the characteristics of natural pozzolans. While chemistry, shape, and fineness are often discussed, there is still a need to understand the role of porosity. One lab experiment showed that the total porosity of natural pozzolans is higher than fly ash, which might mean there is more potential for both pozzolans to absorb batch water. If this is the case, it will be helpful for manufacturers utilizing natural pozzolans to know how quickly and how much, to understand better how batch water is contributing to strength rather than reducing it. In other words: Is there a new water-to-cementitious ratio that can be developed in order to fully realize a repeatable, economical, sustainable, and finishable concrete product using natural pozzolans?
Limestone Calcined Clay Cement
Limestone Calcined Clay Cement (LC3) is a type of cement made by blending limestone, calcined clay, and gypsum. It’s a low-carbon alternative to Ordinary Portland Cement (OPC).
The production process of LC3 involves heating a blend of limestone and clay in a rotary kiln at high temperatures, which causes chemical reactions to take place, resulting in a material known as "calcined clay." This material is then ground into a fine powder and blended with other materials, including limestone and gypsum, to produce LC3 cement.
LC3 has several benefits over OPC, including a lower carbon footprint due to the lower energy requirements during production, improved durability and strength, and a reduced need for clinker, which is a key component of OPC that is responsible for a significant portion of its carbon emissions. It can be produced using locally available materials, which can help reduce transportation costs and support local economies.
History of LC3
The development of LC3 began in the late 1990s when a team of researchers, led by materials scientist Karen Scrivener, began investigating the use of calcined clay as an SCM at the École Polytechnique Fédérale de Lausanne (EPFL) in Switzerland.
In the early 2000s, the EPFL team collaborated with researchers from the Indian Institute of Technology (IIT) Delhi to study the use of calcined clay and limestone in cement production. The resulting blend of limestone and calcined clay was found to have properties comparable to traditional Portland cement but with significantly lower carbon emissions.
Since then, LC3 has been the subject of extensive research and development, with numerous studies conducted to investigate its properties, durability, and environmental impact. In 2016, the Global Alliance for Buildings and Construction (GABC) recognized LC3 as a key innovation for sustainable construction, and the material has since been adopted in several construction projects around the world.
Composition of LC3
The synergetic reaction of limestone with calcined clay in LC3 is a complex chemical process. The result is the formation of new compounds and structures that contribute to the material's properties and performance. Calcined clay contains reactive aluminosilicates, which can react with the calcium carbonate in limestone in the presence of water and cementitious compounds (such as portland cement or clinker) to form calcium aluminosilicate hydrate (C-A-S-H) gel. This gel is a key component of the strength and durability of cementitious materials and contributes to their ability to resist deformation, cracking, and other forms of damage.
The presence of limestone in the mix lowers the amount of clinker, which reduces the carbon footprint of the material. The formation of C-A-S-H gel also results in the consumption of some of the calcium hydroxide produced during the hydration process, further reducing the amount of clinker needed to achieve strength and contribute to durability and sustainability.
LC3 has significantly lower carbon emissions compared to traditional Portland cement, with potential reductions of up to 40-50%. It has comparable or even superior strength and durability compared to Portland cement, making it a viable replacement for conventional cement in many applications. As discussed, it contains a lower amount of clinker, which is the key contributor to carbon emissions in portland cement production. It has been found to improve the workability of concrete, making it easier to place and finish. And, finally, the production of LC3 requires lower temperatures compared to portland cement, resulting in lower energy consumption and reduced emissions from fuel combustion.