A Number and a Story
Learning Objectives:
- Analyze the effects of material choice and design on embodied carbon reduction.
- Use design iterations to determine the best structural design for reducing embodied carbon.
- Have the ability to use facility-specific Environmental Product Declarations in optimizing design.
- Be able to understand how these processes address the SE 2050 and AIA203 environmental commitments.
Credits:
This course is approved as a Structured Course
This course can be self-reported to the AANB, as per their CE Guidelines
Approved for structured learning
Approved for Core Learning
This course can be self-reported to the NLAA
Course may qualify for Learning Hours with NWTAA
Course eligible for OAA Learning Hours
This course is approved as a core course
This course can be self-reported for Learning Units to the Architectural Institute of British Columbia
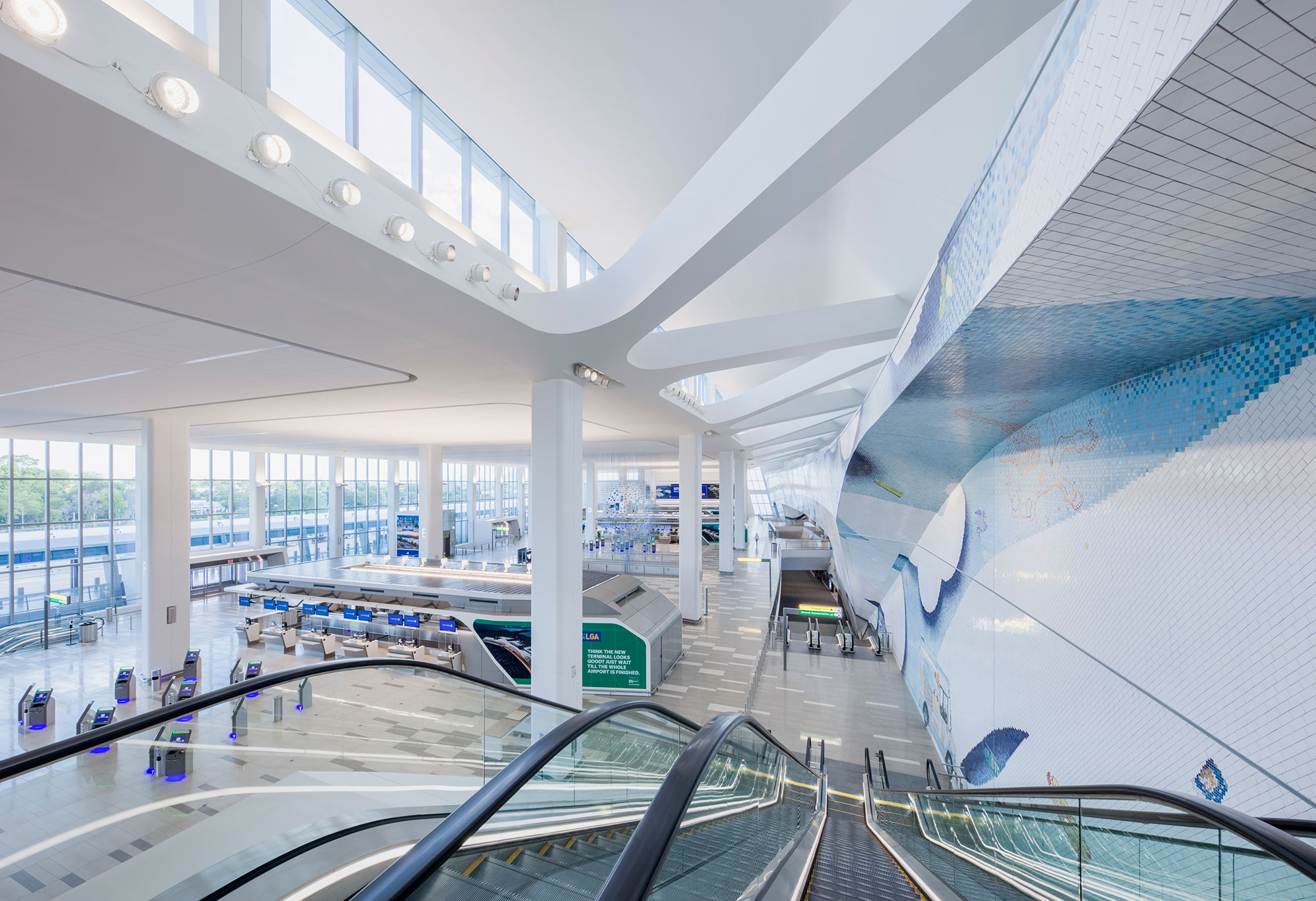
Photo courtesy of LaGuardia Gateway Partners/Jeff Goldberg
LaGuardia Airport’s renovated Terminal B (2022), designed by HOK with WSP, HOK, and Thornton Tomasetti as structural engineers.
As most practicing architects now recognize, decarbonization is an imperative for the profession, the nation, and the planet. Yet decarbonization until recently has been understood as essentially synonymous with reductions in operational carbon emissions, which are only part of the story. That story is now changing as more architects, engineers, clients, and citizens recognize the importance of reducing embodied carbon (EC), and as instruments for estimating it, measuring it, and controlling it become increasingly accessible. EC includes the amount of greenhouse-gas (GHG) emissions associated with the extraction, production, transportation, installation, and end-of-life stage of any product in a building, or of the building as a whole. On the global scale, the manufacture of construction materials and products accounts for 11 percent of annual GHG emissions (EPA 2024), implying significant atmospheric gains from improved performance in this area. Whether carbon accounting is driven by clients’ or other organizations’ sustainability goals, regulatory imperatives, business considerations, or any other type of incentive, reporting and reducing EC is an essential aspect of contemporary design and construction. Decarbonization efforts also involve a proliferation of benchmarks, complicating communication and collaboration among architects, engineers, contractors, and clients.
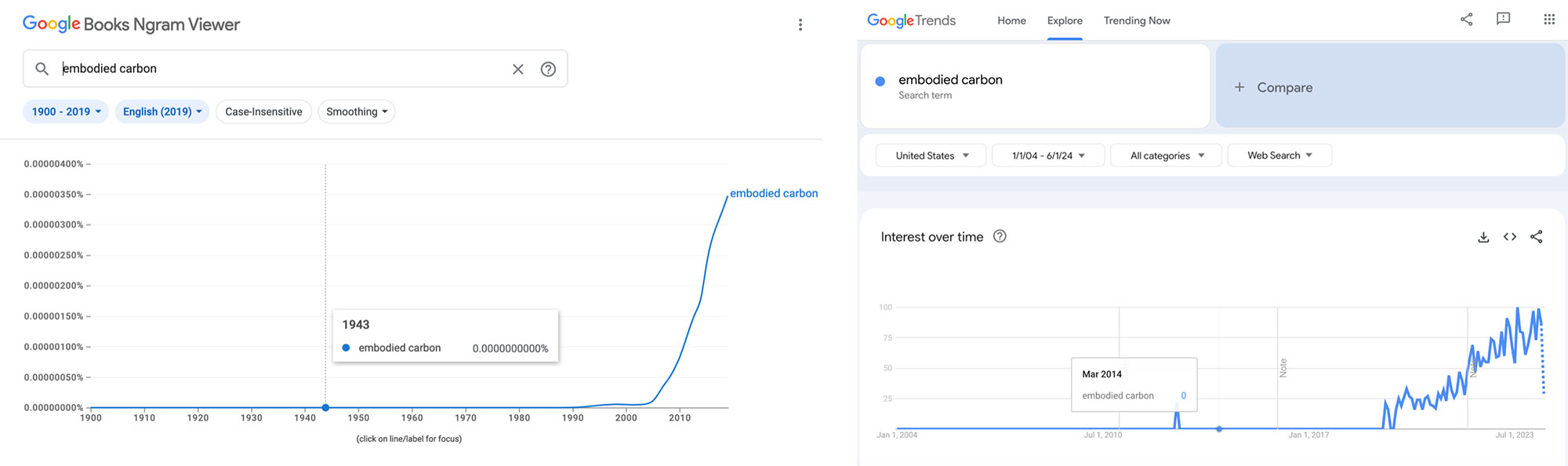
Images courtesy of author’s screen captures, June 1, 2024
Figure 1A (left): Google Books Ngram Viewer data since 1900. Figure 1B (right): Google Trends data since 2004 on the frequency of the phrase “embodied carbon” in books published in English and in online searches.
Awareness of EC as well as operational carbon, says Max Driscoll, AIA, LEED-AP, vice president for sustainability at AECOM Tishman in New York, has taken the shape of “a hockey-stick curve. It was basically zero five years ago, and it’s really going up and up.” (Measurements of the use of the phrase “embodied carbon” using Google Books Ngram Viewer over the past century and Google Trends for online searches over the past 20 years bear out Driscoll’s assessments; see Figures 1A and 1B.) Accounts of attention to EC, like the familiar hockey-stick curve of geophysicist Michael Mann and colleagues showing global temperature rising sharply beginning with the 20th century (Mann et al. 1999), involve nuances and margins of error, yet Driscoll and other commentators describe EC as a critical component of contemporary practice, an area where recognized strategies can combine with ample low-hanging fruit to yield substantial environmental benefits.
Luke Johnson, sustainability specialist at the steelmaker Nucor, notes that operational carbon is intuitively easier to grasp, while EC presents more of a learning curve. “I think it’s easy to understand heating and cooling costs or electrical costs and riding a bike to work versus driving your car; those are things that you can relate to any building. Now, when you talk about a steel building or a concrete building or a wood building, the supply chains there are dramatically different, and therefore the embodied carbon in that supply chain is harder to understand, because it’s not an apples-to-apples comparison between materials.... A full life-cycle assessment is what you really have to do to fully understand the embodied carbon, and that’s another added kind of time and cost.”
The building and construction industries in general, according to Carbon Leadership Forum (CLF) figures taken from the United Nations Environment Programme and International Energy Agency’s joint 2017 report, account for nearly 40 percent of global energy-related carbon dioxide emissions in constructing and operating buildings, including the impacts of upstream power generation (CLF “Climate”). Ironmaking and steelmaking account for 7-8 percent of annual global carbon emissions (IEA), and about half of all steel produced is for the construction sector, placing direct influence on about 4 percent of global carbon emissions in the hands of structural engineers using steel. Since U.S. building codes mainly address operational carbon, EC reporting and management are a professional responsibility.
Efforts to reduce EC “gained a lot more traction earlier on the West Coast,” says Michael Cropper, P.E., LEED AP, associate principal at Thornton Tomasetti, DC Structures Practice Leader in Washington, DC, and co-leader of the firm’s Embodied Carbon Community of Practice. Interest in EC, Cropper says, is particularly high in larger markets, among institutional clients with “strong ESG [environmental, social, and governance] and sustainability goals,” and at larger architecture and engineering firms with the resources to perform the necessary research. There are costs associated with managing information about EC, and “because it’s a new topic, there’s a sense of inherent risk,” Cropper continues, yet “what we have seen is a lot more acceptance.... Once in a market, you start to see a successful project implement these kinds of reductions and gain traction from leasing or good press.” The LEED system, currently in version 4.1, addresses EC in its Materials and Resources credits and will give up to four LEED points for EC assessment and reduction in the forthcoming version 5 of the program, expected to appear in 2025. State and federal Buy Clean initiatives create similar incentives to keep EC below designated thresholds in publicly supported projects.
Allan M. Paull, senior vice president for civil and structural engineering at AECOM Tishman, emphasizes three criteria for evaluating measures to reduce EC. “Whatever solutions you have have to work, have to be sustainable from a building standpoint, and have to be cost-effective.” Clients’ commitment to EC reduction, he notes, is also quantifiable: “Maybe they’re willing to pay 2 or 3 percent more; no one’s willing to pay 50 percent more to be environmentally sensitive.” Paull cites environmental/energy scientist Vaclav Smil’s 2022 book How the World Really Works for a sobering, factually rigorous explanation of processes involved in the transition to cleaner energy, which Smil says is contingent on deliberate human choices (rejecting determinism in the direction of either “catastrophism” or “techno-optimism” [Smil 9]) and unlikely to proceed as swiftly as many would prefer. The four key material ingredients of civilization that have allowed the world population to grow to 8 billion people, Smil asserts, are steel, concrete, ammonia, and plastics. “Steel and concrete,” Paull says, are thus “two of the four foundations of society that allow the planet to support way in excess of what it would support without that.”
The steel industry, at least in the United States, has moved decisively toward circular manufacturing processes over recent decades (see “Structural Steel for Low-Carbon-Emission Lightweight Frames,” Architectural Record, March 2024); the concrete industry has further to go in this regard, commentators suggest, and may offer opportunities for considerable improvement if new decarbonization processes prove scalable. Since “56 percent of our carbon footprint is tied up in those two trades,” as Paull’s colleague Driscoll points out, design teams and structural engineers working with those two materials are in a position to make substantial progress through detailed attention to the EC associated with structural components.
PROLIFERATING TOOLS AND THEIR BEST USE
Multiple organizations and programs have offered guidance to professionals seeking to understand and control EC, including the CLF, the American Institute of Architects’ AIA 2030 Commitment (and its Design Data Exchange), the Structural Engineering Institute (SEI)’s SE 2050 Commitment, the Associated General Contractors of America (AGC), the Aluminum Extruders Council, the Urban Land Institute, the Rocky Mountain Institute, the U.S. Environmental Protection Agency (EPA), and others. The World Resources Institute’s GHG Protocol sets standards for direct (Scope 1) and indirect (Scopes 2 and 3, defined by positions in the value chain) emissions and provides guidance on reduction strategies tailored to different economic sectors. The CLF maintains a useful list of tools for whole-building life-cycle assessments (LCAs) and calculators for individual materials or assemblies, from the Embodied Carbon in Construction Calculator (EC3), a widely used free database of Environmental Product Declarations (EPDs), to more product-specific tools and proprietary software programs, including OneClick, Tally, Athena, the Buildings and Habitat Object Model (BHoM), Build Carbon Neutral, Early Phase Integrated Carbon (EPIC), and many more.
The AGC’s Decarbonization and Carbon Reporting Playbook provides contractors with step-by-step recommendations to be used alongside online emissions calculators, eight of which are linked at the AGC website; the Playbook is free, not hidden behind a paywall (an advantage for small to medium-sized firms, Driscoll comments). CarbonCare, created by a not-for-profit logistics organization based in Engelberg, Switzerland, allows input of shipment methods and routes, calculating the EC associated with multimodal transportation options and informing decisions about the relative GWP of local or global material sources. SE 2050 includes Embodied Carbon Action Plans that focus on four areas, extending beyond single project-level concerns: education, reporting, advocacy, and reduction. Thornton Tomasetti, Cropper notes, was a co-founder of the original SE 2050 Challenge and a founding signatory of its successor the SE 2050 Commitment; as of this writing, the firm has submitted 65 projects to the SE 2050 database, and its R&D studio CORE has developed its own Revit plugin for EC takeoffs and Global Warming Potential (GWP) calculations, Beacon, an open-source program free for anyone’s use.