Reducing Embodied Carbon in Concrete
No Impact on Cost or Performance
The use of both OPC and PLC has been researched, developed, and documented by industry-wide trade associations for both buildings and highways. The result of that work has been the development of standard specifications and testing requirements for different types of concrete. The PCA points out that “To ensure a level of consistency between cement-producing plants, certain chemical…limits are defined by a variety of standards and specifications. For instance, Portland cements and blended hydraulic cements for concrete in the U.S. conform to the American Society for Testing and Materials (ASTM) C150 (Standard Specification for Portland Cement), C595 (Standard Specification for Blended Hydraulic Cement) or C1157 (Performance Specification for Hydraulic Cements).”
The referenced ASTM C150 describes the five standard types of Portland cement including:
-
Type I Normal
Type II Moderate Sulfate Resistance
Type II (MH) Moderate Heat of Hydration (and Moderate Sulfate Resistance)
Type III High Early Strength
Type IV Low Heat Hydration
Type V High Sulfate Resistance
Specifying one of these particular types of Portland cements to be used in the concrete for a building means specifying the corresponding mix of ingredients to achieve the designated characteristics of each type.
Moving to blended hydraulic cements, (i.e., those that introduce other ingredients beyond the ones in pure Portland cement) those specifications are covered in a different standard—ASTM C595 “Standard Specification for Blended Hydraulic Cements.” This is intended for use on all applications including buildings, stating “This specification pertains to blended hydraulic cements for both general and special applications, using slag or pozzolan, or both, with Portland cement or Portland cement clinker or slag with lime.” In this case, the standard defines four additional types of cement based on the nature of the blended ingredients:
- Type IL Portland-Limestone Cement
Type IS Portland-Slag Cement
Type IP Portland-Pozzolan Cement
Type IT Ternary Blended Cement
The Type IL Portland-limestone cement specification prescribes ingredients and proportions with some performance requirements. An alternative is to use the performance-based specification ASTM C 1157/C 1157M which is a blended cement specification where performance criteria alone govern the products and their acceptance.
For site work and paving concrete, the American Association of State Highway and Transportation Officials (AASHTO) has addressed the use of PLC in its standard AASHTO M 240, “Standard Specification for Blended Hydraulic Cement” (updated in 2021). It states that “This specification pertains to blended hydraulic cements for both general and special applications, using slag, pozzolan, limestone, or some combination thereof, with Portland cement or Portland cement clinker or slag with lime.”
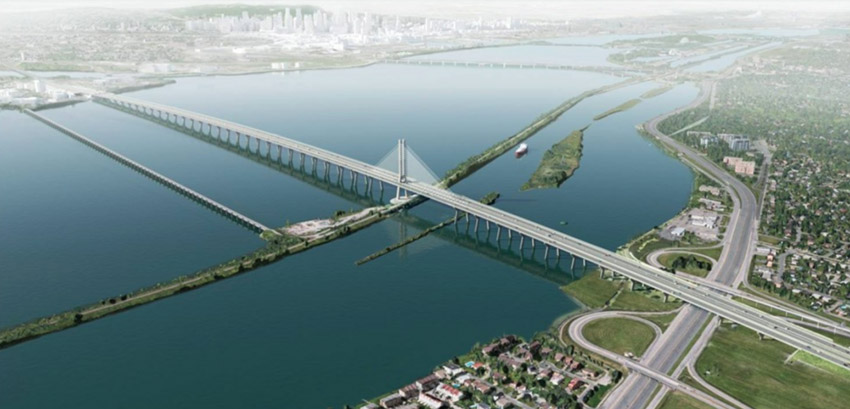
Photo courtesy of Holcim
Type IL Portland-limestone cement can be specified in highway and bridge construction as well as in buildings to reduce embodied carbon.
All of these specifications prescribe the nature of the ingredients and refer to standard test methods to assure that the testing is performed in the same manner. That means that all of the performance criteria for the fundamental strength and durability of the cement, whether OPC, PLC, or other blended cements, are still assured to be achieved. For the designer and specifier, that means that there is no difference in the performance of Type I OLC compared to Type IL PLC.
It is also worth noting that increasing the amount of limestone in PLC is offset by reducing the cost of other ingredients in OPC. Additionally, the general process for creating PLC is essentially the same as the process for OPC. Therefore, cement manufacturers report that there is virtually no cost difference in concrete that uses Portland-limestone cement compared to comparable concrete mixes using ordinary Portland cement.
Supplementary Cementitious Materials (SCMs)
Other types of blended cements are covered in the standards beyond PLC. However, while PLC simply increases one ingredient (limestone), the other types of blended cements are based on different ingredients being added. Those other ingredients are referred to as supplementary cementitious materials (SCMs) and include tested materials with known characteristics such as slag cement, fly ash, silica fume, pozzolans, or metakaolin. These SCM specifications allow for the reduction of Portland Cement clinker by substituting some of it with prescribed amounts of the appropriate SCM(s). When used properly, SCMs do not diminish concrete performance, rather, they are usually selected to improve performance in specific ways including:
- Enhanced strength
- Reduced permeability
- Resistance to alkali-silica reaction (ASR)
- Resistance to sulfate attack
- Resistance to thermal stress
Keep in mind that PLC is not an SCM, rather, it is a different type of blended cement. That means that SCMs can be used in either OPC or PLC to achieve the desired performance enhancements and embodied carbon reduction. For all the specifications of the different types of blended cements, the same amount of SCMs can be added to either OPC or PLC. Therefore, the combination of using PLC and SCMs together has the potential for the greatest possible reduction in embodied carbon since the combination reduces more clinker than either one does individually.
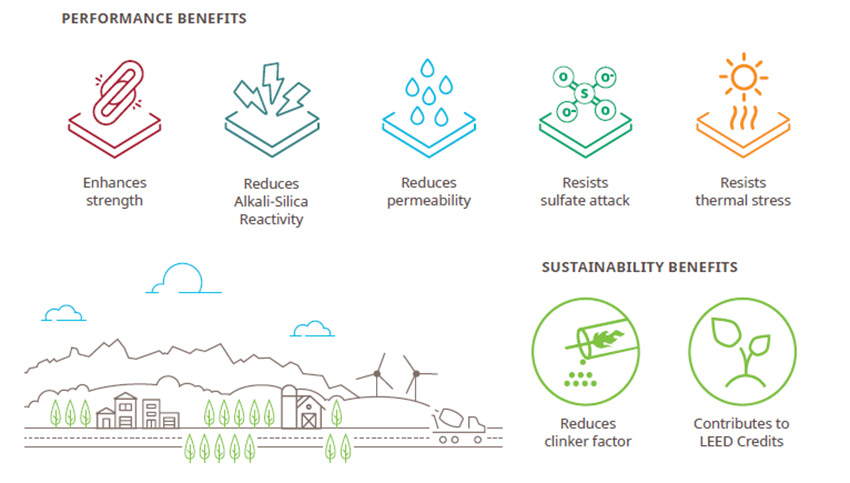
Photo courtesy of Holcim
Type IL cement combined with SCMs can produce enhanced capabilities in terms of performance and sustainability.