Concrete Impacts
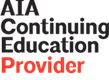
Learning Objectives:
- Recognize how concrete can reduce the life-cycle impacts of a building.
- Define life-cycle assessment (LCA) and explain how it can be used to help measure and reduce the environmental impacts of a building.
- Discuss how LCA is used in the green building standards.
- Describe an example of how LCA software tools can be used to reduce the environmental impact of a building, including carbon footprint,0920026003 GBCI
This course is part of the Concrete Academy
Enhanced Concrete
Enhanced concrete is specially designed concrete for particular uses, from large civil engineering projects like bridges and dams to thin concrete structures in residential projects. Some examples are year-round, high-early-strength, self-consolidating, and high-performance, among others. These concrete technologies create more strength, rigidity and durability characteristics, which allows them greater longevity and can cut back on other emission sources related to construction timelines. Enhanced concretes can be specified for low-carbon options, with optimized mixes that use positive properties of SCMs.
RESEARCH ON LCA FOR CONCRETE
Though more assessments have been done in recent years, there are still relatively few examples of whole-building LCAs in the literature. LCA is still a relatively new science for the purposes of measuring environmental impacts of buildings and can be extremely time consuming and expensive. Most researchers have only conducted partial LCAs and choose to limit the scope of an LCA by ignoring certain life-cycle stages because of the lack of data or scope of research. Others focus on specific impacts to simplify the LCA process. The following are several examples of research that compare the life-cycle impacts of concrete, steel, and wood-framed buildings.
A comprehensive whole-building LCA study was conducted at MIT and published in a report titled Methods, Impacts, and Opportunities in the Concrete Building Life Cycle. The research explores and advances key areas relevant to the field of whole building LCA, including methodology, benchmarking, and impact reduction. In particular, the research compared the environmental impacts of a concrete building to a steel building for a large commercial structure in two different climate zones and of single and multifamily structures built with concrete and wood-frame in two different climate zones.
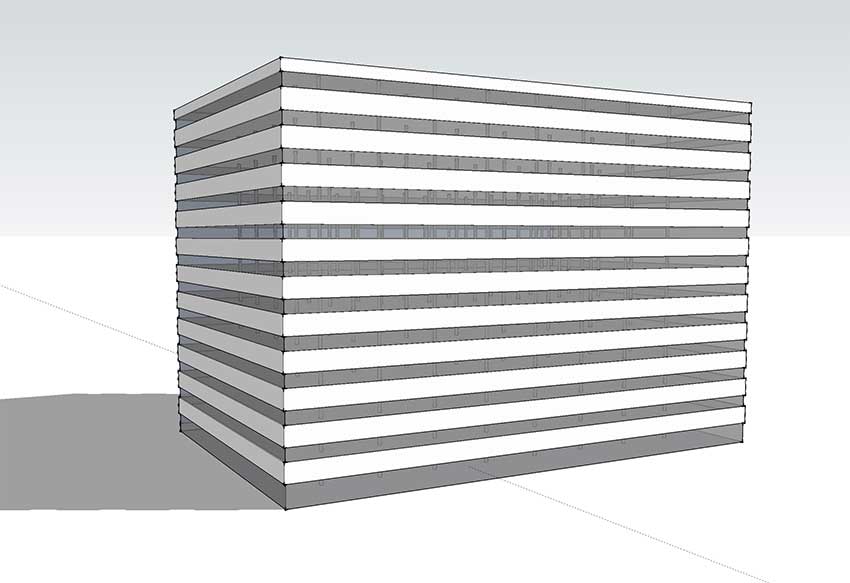
Image courtesy of NRMCA
Figure 5: Twelve-story commercial building with 40% glazing and 60% aluminum panels studied at MIT.
LCA of a Commercial Building
The comprehensive LCA was conducted for a 12-story, 46,321-square-meter (498,590-square-foot) commercial building (Figure 5). The building was analyzed for two climates, Phoenix and Chicago, and for two different structural materials, concrete and steel. The annual operating energy, determined using building energy analysis software, was conducted for a 60-year life cycle. The Global Warming Potential (GWP) was quantified using CO2 equivalents (CO2e) for several purposes, including benchmarking emissions for current construction practices, comparing impacts of concrete versus steel, and understanding the relative magnitude of impacts for different life-cycle stages.
The analysis demonstrated that the greenhouse gas emissions due to operational energy of the benchmark buildings are responsible for 95-96% of life-cycle emissions. Compared to the steel structure, the concrete building has approximately the same embodied emissions (pre-use, maintenance, and end-of-life phases) but have lower operating emissions (use stage).
The LCA research drew several conclusions, including:
The concrete and steel commercial buildings studied had similar embodied GWP of 42 lbs CO2e/ft2 (205 kg CO2e/m2); Thermal mass of an exposed concrete frame can provide HVAC savings of 7-9% compared to a steel frame. This accounts for 2% savings in annual operating emissions; Over a lifetime of 60 years, the CO2e emissions of the concrete building were slightly lower than the steel alternative (see Figure 6).
Finally, several recommendations for reducing life-cycle emissions of concrete buildings were presented. In particular, the GWP reduction effects of SCMs in concrete, such as fly ash, were quantified. Additional options for reducing operating emissions were introduced and quantified within the full life cycle. There are a number of potential emissions reduction strategies for concrete buildings and LCA provides guidance for future environmental improvements, including:
- Increasing SCM substitution (such as fly ash) in the concrete building from 10% to 25% can decrease pre-use GWP for the building by 4.3%.
- Low-lift cooling can decrease the operating energy requirements for concrete buildings since low-lift cooling takes advantage of the high heat capacity of concrete.
LCA of a Single-Family and Multifamily Building
The same general methodology was applied to structures used in residential applications. LCA was conducted for a two-story, 2,400-square-foot (223-square-meter), single-family building and a four-story multifamily apartment building with a total square footage of 33,763 square feet (3,137 square meters), as shown in Figure 7. The concrete buildings were designed using Insulated Concrete Form (ICF) construction consisting of concrete walls encased in expanded polystyrene (EPS) insulation. The wood buildings were designed using typical light-frame wood construction. For all buildings, the roof, partitions, and floors are designed in the same manner. Design of the exterior walls and foundations vary between the different buildings.
The LCAs were carried out for two different cities in the U.S. to model regional and climatic differences―Chicago, representing a cold climate, and Phoenix, representing a hot, dry climate. The annual operating energy, determined using energy analysis software, was conducted for a 60-year life cycle.
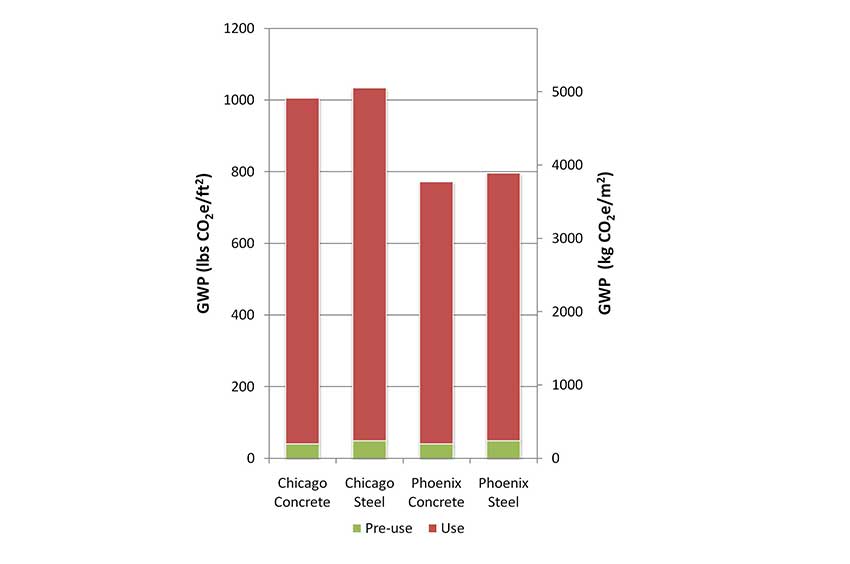
Image courtesy of NRMCA
Figure 6: Total global warming potential (GWP) over 60-year life span for a commercial building.
The resulting Global Warming Potential (GWP) was then quantified using CO2-equivalents (CO2e) for a number of purposes, including benchmarking emissions for current practices, comparing concrete with wood, and understanding the relative importance of different life-cycle stages. In particular, the research demonstrates that there are measureable differences between various construction materials and that concrete structures can provide unique benefits compared to other materials over an operating life cycle.
According to the report, concrete homes have a higher embodied GWP in the pre-use stage, but this stage accounts for only about 2-12% of the overall GWP for the life of the home. For a cold climate, such as Chicago, the energy savings of an ICF house built from average to-tight levels of air infiltration saves 23% of total operating energy; and over a 60-year life cycle, the lower operating GWP of the concrete buildings outweigh pre-use emissions resulting in 5-8% lower GWP for single-family and 4.4-6.2% lower GWP for multifamily (see Figure 8).
The MIT study offered a number of opportunities for reducing GWP of concrete residential buildings:
Moving from a 6-inch (15.2 cm) to a 4-inch (10.2 cm) concrete core is both cost-effective and reduces emissions; using ICFs with thicker insulation panels, increasing air tightness, and using thinner concrete walls can reduce GWP; and increasing SCM substitution from 10% to 50% in the ICF walls reduces the pre-use GWP by 12% to 14%.
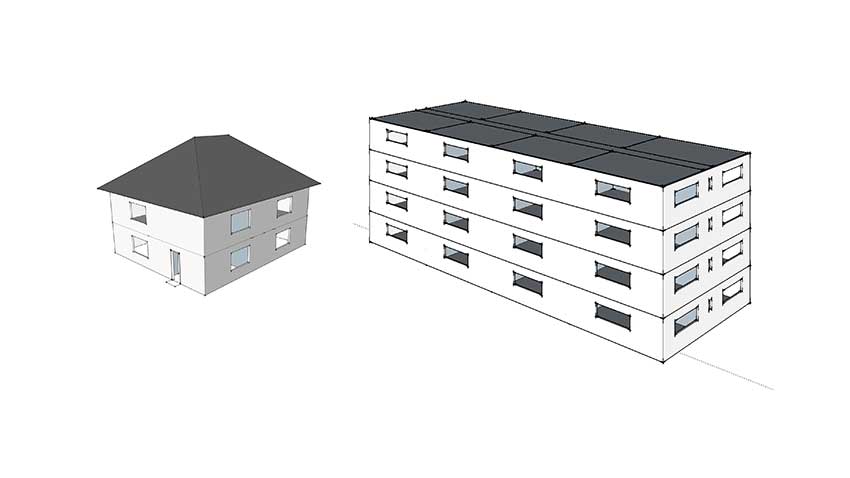
Image courtesy of NRMCA
Figure 7: Two-story, single-family and four-story, multifamily buildings studied by MIT.
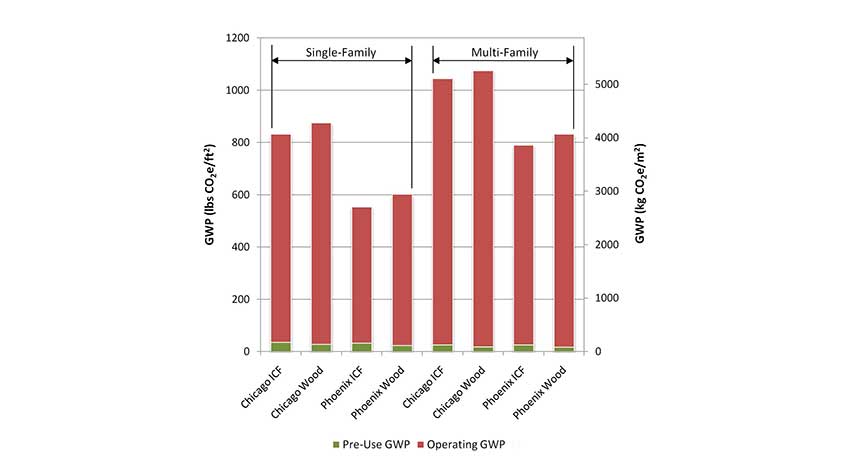
Image courtesy of NRMCA
Figure 8: Pre-use and operating GWP over 60-year life span for single-family and multifamily residential buildings.