Concrete Impacts
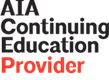
Learning Objectives:
- Recognize how concrete can reduce the life-cycle impacts of a building.
- Define life-cycle assessment (LCA) and explain how it can be used to help measure and reduce the environmental impacts of a building.
- Discuss how LCA is used in the green building standards.
- Describe an example of how LCA software tools can be used to reduce the environmental impact of a building, including carbon footprint,0920026003 GBCI
This course is part of the Concrete Academy
How Can Concrete Help Reduce Life-Cycle Impacts of a Building?
Assessing all of the building materials in a project is a complex task. The best approach to reducing carbon impacts is starting with the products that have the biggest immediate impact: concrete, steel, and aluminum. When it comes to concrete, portland cement is responsible for most of the carbon emissions—roughly 90%. Reducing manufacturing process energy and identifying the places where high-emissions ingredients can be reduced and substituted, is one of the best approaches for addressing life-cycle impacts of a building.
Reduced Manufacturing Process Energy
Water, sand, stone, gravel, and other ingredients make up about 90% of a concrete mixture by weight. The process of mining sand and gravel, crushing stone, combining the materials in a concrete plant, and transporting concrete to the construction site, requires very little energy and therefore only emits a relatively small amount of CO2 into the atmosphere. The amount of CO2 embodied in concrete is primarily a function of the cement content in the mix.
Therefore, one of the most effective ways to reduce the embodied impacts of concrete manufacturing is to increase the use of industrial byproducts, such as fly ash, blast furnace slag, and silica fume, to supplement a portion of the cement used in concrete. These industrial byproducts, which would otherwise end up in landfills, are called supplementary cementitious materials or SCMs for short. SCMs work in combination with portland cement to improve strength and durability of concrete. In addition, their use reduces the CO2 embodied in concrete by as much as 70%, with typical reductions ranging from 15−40%, depending on the percentage replacement of portland cement.
Concrete producers also incorporate a variety of environmental best management practices in the production of concrete. These include the reuse and recycling of waste from concrete manufacture, such as water and unused returned concrete. It also incorporates waste byproducts from other industries such as recycled industrial wastewater, foundry sands, glass, and other materials that would typically end up in landfills. In addition to the use of SCMs in the concrete mix, concrete from demolition can be crushed and recycled as aggregate. Recycled aggregate is often used as backfill and pavement base and is sometimes used for making new concrete. Reinforcing steel in concrete, which is made from recycled materials, can be recycled and reused.
Portland-Limestone Cement
When narrowing down the carbon emissions in cement, the issue really comes down to clinker. The solid intermediate material produced in the manufacturing portland cement is created by sintering limestone and aluminosilicate materials, such as clay, during the kiln stage. That product is where most of portland cement’s emissions occur: About .90 to .92 tons of C02 can be associated with 1.0 ton of clinker. Increasing kiln efficiency by using dry instead of wet kilns is one way to reduce C02. The other way to reduce the clinker impacts lies in the processing and chemical formulation of the cement and in assessing the proportion of ingredients used.
As the cement industry pursues carbon reduction, acceptable alternatives to ordinary portland cement have surfaced. One of the most promising and immediate options is changing OPC, classified as Type I cement, to portland-limestone cement (PLC) classified as Type IL cement. PLC contains more ground limestone and can replace the amount of clinker required to be used in the cement. Ordinary portland cement is limited by definition and standards to a maximum of 5% limestone. Portland-limestone cement, on the other hand, is allowed to contain 5−15% limestone.
Limestone is softer than clinker and grinds preferentially. To effectively achieve equivalent performance, PLC is typically ground to a higher fineness than portland cement. While there is some evidence that limestone reacts chemically, the contribution to strength from chemical reactions is marginal. It is postulated that finer limestone particles improve particle packing in concrete (similar to fine SCMs) and provide reaction sites for more efficient hydration of cement. There is considerable research that compares and documents the equivalent performance of concrete made with PLC to those made with portland cements. These include the effects on fresh concrete, hardened concrete properties like strength and shrinkage, and, for impacts on durability such as freeze-thaw resistance, alkali-aggregate reactions and other properties.
An LCA report conducted by Athena Sustainable Materials Institute3 compared the GWP of a typical 5000 psi (35 MPa) concrete made with ordinary portland cement (OPC) and a PLC composition. Both concretes had identical mixture proportions, including the cementitious component. According to the Athena report, the GWP or carbon footprint of the concrete made with OPC is 299 kg CO2/m3 compared to 272 kg CO2/m3 for concrete made with PLC, which is a 9% reduction. PLC with 15% limestone would reduce carbon footprint further.
The use of both OPC and PLC has been researched, developed, and documented by industry-wide trade associations for both buildings and highways. The result of that work has been the development of standard specifications and testing requirements for different types of concrete, including the acceptance of Type IL cement in state specifications. ASTM C595 and the use of Type IL is accepted in the ACI 318, Building Code for Structural Concrete, ACI 301, Specification for Structural Concrete, ASTM C94, Specification for Ready Mixed Concrete and the AIA MasterSpec that is used by design firms to develop their specifications for private projects.
Approximately 100 million tons of cement are produced annually in the U.S., but only about 2 to 3% is currently specified and sold as PLC. Architects and other design professionals can help by changing their concrete specifications and including to Type IL PLC (ASTM C 595). Switching helps to move the entire construction industry toward environmentally responsible action. Industry wide, it is estimated that the switch to PLC could reduce energy consumption by 11.8 trillion Btus, and carbon dioxide emissions by more than 2.5 million tons, per year.
Durability, Thermal Properties, and Enhanced Concretes
Concrete structures can help reduce life-cycle impacts of buildings even after the manufacturing process and beyond construction. For instance, concrete structures withstand the test of time and, as a result, have low environmental impacts associated with maintenance and repair. In addition, concrete’s thermal- mass qualities reduce energy use throughout the life of the building.
Durability
For a building to last for generations, durability must be an inherent quality of its construction materials. Concrete does not rust, rot, or burn. Concrete buildings are durable, long-lasting structures that resist natural disasters such as hurricanes, tornadoes, earthquakes, fires, and floods. Because of its longevity, concrete is a viable solution for environmentally responsible design over the lifetime of a building. As a result, concrete buildings have low environmental impacts associated with maintenance and repair.
Thermal Mass
In a building, it is the mass of the building elements that stores heat during the hottest periods of the day and releases the heat during the cooler evening hours. Concrete is one of few building materials that possess thermal-mass properties. In the winter season, high-thermal-mass concrete walls and floors absorb radiant heat from the sun and gradually release it back into the occupied space during the night when the outdoor temperature drops.
High-thermal-mass buildings have distinct benefits. Reduction takes place through moderate shifts in peak loads of energy requirements due to the reduction in high fluctuations between indoor and outdoor temperatures. Heat transfer through a high-thermal-mass wall is reduced, and therefore less energy is used to heat and cool the interior space. The thermal mass of concrete delays peak temperatures, and reduces and spaces out peak energy loads, therefore shifting the energy demand to off-peak periods when utility rates may be lower.