Meeting Energy Demands While Facing the Challenges of Electric Grid Instability
Carbon Intensity
Given the current reliance on fossil fuels, a discussion of the electric grid should also include a look at carbon intensity, which is a measure of how much carbon dioxide (CO2) and other greenhouse gases are produced per unit of activity. It can be used to compare the carbon impacts of electricity, transportation fuels, or the production of a product. Most commonly, it refers to how many grams of carbon dioxide (CO2) are released to produce a kilowatt hour (kWh) of electricity. Obviously, electricity that’s generated using fossil fuels is more carbon intensive, since the process by which it’s generated creates more CO2 emissions than renewable sources.
When comparing large-scale energy usage across the grid and different fuel sources, the common energy measurement used is a joule which is equal to 1 watt of electricity per second. Hence, 1,000 watts generated in one second, (i.e. 1 kilowatt) is equal to 1,000 joules. However, one kilowatt hour (kWh) is by definition, 3,600 seconds of energy. Therefore, the conversion of kilowatt hours to joules means that 1 kWh = 3,600,000 joules. When dealing with larger amounts of kWh, it is useful to talk in terms of megajoules (MJ) which is simply a million joules – so 3,600,000 joules is 3.6 megajoules. Equivalent calculations and conversions can be done for other fuels too, to determine the number of megajoules in those fuels.
The corresponding information on the amount of energy produced is the amount of carbon dioxide (CO2) or its equivalent that is emitted during the energy generation process. That is typically calculated as a measurement of grams. When data from different energy sources are used to determine the carbon intensity, it is typically expressed as grams of CO2 generated (or equivalent) for each megajoule of energy produced—expressed as gCO2e/MJ.
When this process is used on the electrical grid, we can get a sense of the carbon intensity of the grid compared to other fuels. Since different parts of the utility grid can access electricity created with different fuel sources, there are different carbon intensity scores that can be calculated for each state in the country. When these are averaged together the electrical grid has a comparatively high carbon intensity. Considering the various source energies used, the U.S. average carbon intensity score is around 130. By comparison, petroleum and diesel score around 91, while natural gas and propane range from 71-79. Renewable propane scores as low as 7 to 43.5.
By understanding the true nature of carbon intensity, it is easier to discern which sources actually contribute to decarbonization today and which ones still aren’t there yet. The use of alternative energies, like propane, provides lower carbon intensity now and offers the potential of even greater reductions as more renewable propane becomes available.
Costs Based on “Spark Spread”
All purchasers of energy usually want to know what the cost of that energy is and how it compares to other energy sources. When different types of fuels or energy sources are used, then some type of conversion needs to be done to compare the same energy output—the price of a million watt-hours (i.e., a megawatt-hour (MWh)) is not the same as the price of a million Btu (mmBtu) nor do they have an equivalent amount of energy. Therefore, in order to do an “apples to apples” comparison of the cost of using electricity compared to using propane, a conversion calculation is necessary. That calculation is referred to as the “spark spread” and is defined simply as the price difference in a particular market area between electricity generated by the local utility and that of propane fuel for equivalent amounts of energy.
In order to calculate the spark spread for a particular project, first the power price or cost of electricity per Megawatt hour ($/MWh) is needed—generally as published by the utility company. Then the propane cost is identified in terms of cost per million Btu ($/mmBtu)—generally available from a propane supplier. The propane cost is then multiplied by a conversion factor based on the heat rate of propane compared to electricity—i.e., the number of Btus per megawatt hour (mmBtu/MWh). The cost of the propane can then be expressed in cost per megawatt hour ($/MWh) and be compared to the electricity cost per megawatt hour ($/MWh). The difference between the two numbers is the “spark spread” and can be used to determine a fair comparison of what is really being paid for energy between the two energy sources.
In some market areas, where electricity is available from the grid at a higher price, then the spark spread of propane or other fuels can be quite favorable, meaning propane is more economical. If electricity is a lower price than propane, the spark spread may not be favorable. Of course, calculations are typically done only at current electricity rates. It is generally held that electric rates are expected to continue to increase as demand increases and improvements are needed for the grid. In that light, it would be easy to compare the projected spark spread if rates go up during the life of a propane system and determine the “crossover” point where propane becomes more favorable. Ultimately, the building owner or operator can use this information to determine which is the most economically favorable solution and a system can be designed accordingly.
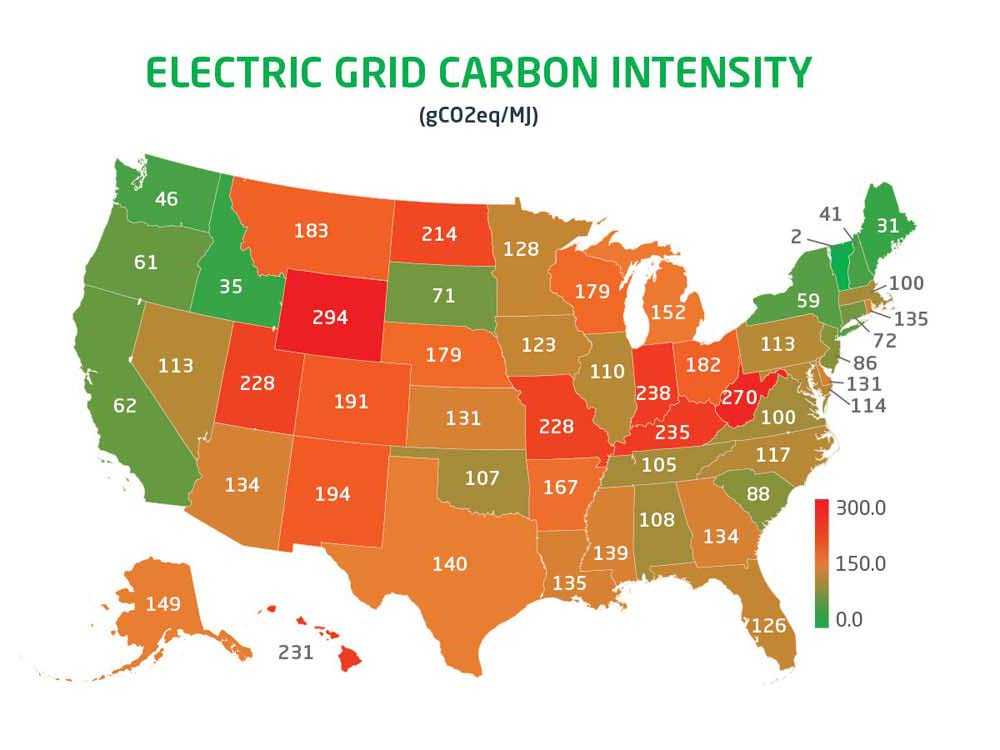
Photo courtesy of Propane Education & Research Council
The carbon intensity of the electrical grid varies by state but is nonetheless rather high since coal and natural gas are still the primary fuels used for electrical generation.
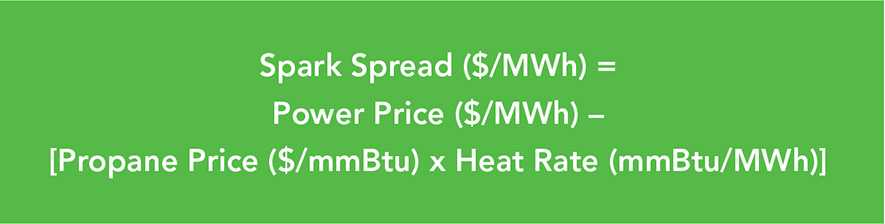
Image courtesy of Propane Education & Research Council
The Spark Spread for a particular project is calculated as the cost difference between electricity from the grid and locally produced energy using propane.
PROPANE ENERGY SYSTEMS
Keeping in mind all of the foregoing, propane can readily be used in buildings either as a stand-alone fuel source or as part of a hybrid energy strategy. As a stand-alone solution, it has often been used in locations that don’t have natural gas available (i.e. rural or edges of metropolitan areas). Lately, it has also been used where natural gas usage is being restricted by local regulations for environmental protection and/or in the interest of reducing fossil fuel use. In both of those cases, propane can help serve these locations with cleaner solutions for space heating, hot water production, cooking, or other residential and commercial uses. In order to help compare costs, even beyond the spark spread, most equipment manufacturers have an economic impact calculator to review the cost-benefit of using their product and the corresponding reduction in grid demand.
In addition to conventional space heating and appliances, there are also innovative propane-powered products available like Combined Heat and Power (CHP), propane cooling, power generation, and even snow melt systems to provide safety and comfort and ensure continuous building operations. Such ongoing operations help building owners limit their liability in the event of power outages—often a genuine economic advantage.
Since propane has been in use for a long time in this country, there are comparable codes and standards for propane equipment just like any other mechanical equipment used in buildings. Propane equipment manufacturers hold certifications for performance and quality control from recognized testing organizations such as UL, CSA, ETL, AHRI, etc. Local and national building codes (e.g., the International Construction Codes – ICC) recognize propane as a fuel source and it can be readily used to meet safety and energy efficiency requirements in those codes. All of this points to the fact that when safe, proven, and cleaner energy solutions are the challenge, propane is a viable solution.
In the following sections, we explore some of the specific energy systems that are being successfully used in buildings and facilities of all types.
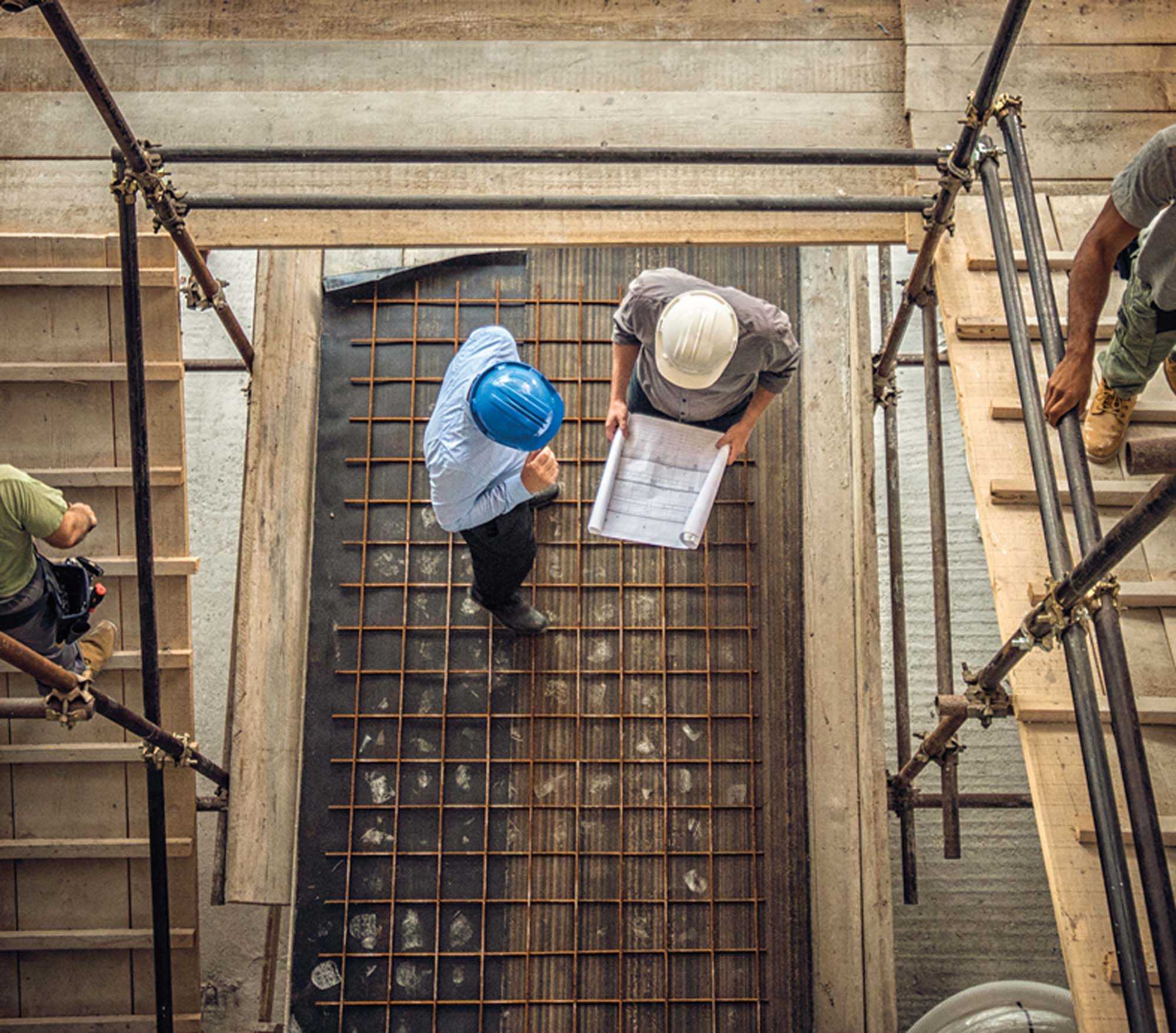
Photo courtesy of Propane Education & Research Council
The economics of using propane can be assessed for every building project.
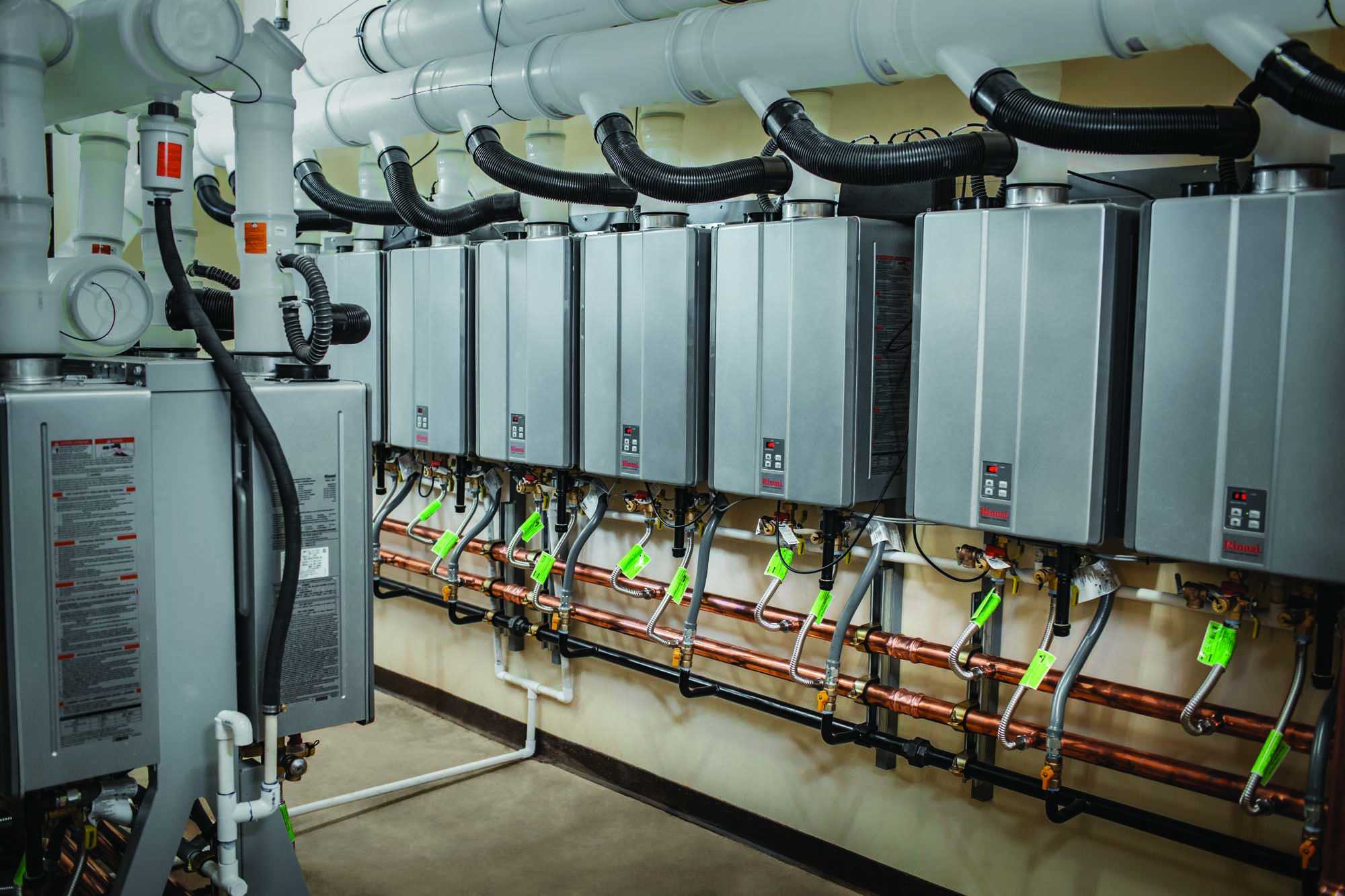
Photo courtesy of Propane Education & Research Council
Hybrid systems effectively combine on-site propane-fueled components with other building mechanical systems.
Hybrid Systems
While propane can be used as a stand-alone system, it is also possible to use it as part of an overall hybrid energy strategy. This can allow building electrical systems to be more appropriately sized and reduce total costs. For example, a recent innovation in hydronic heating systems is to use a propane tankless water heater that is attached to an electric heat pump to replace the need for electric resistance backup heating in colder temperatures. This is accomplished by the use of a hydronic coil custom fit to match a heat pump air handler plus a circulating pump and a control module. The pump moves hot water from the tankless water heater to the hydronic coil, then the heat pump air handler fan blows air across the hydronic coil to heat the building. The results of this type of system commonly include:
- Improved comfort since hybrid heat pump solutions can deliver heat faster and more consistently than all-electric systems.
- Greater affordability compared to all-electric systems—hybrid heat pump solutions are cheaper to run than all electric systems using electric resistance backup heating. They have been shown to reduce operating costs by up to 35 percent.
- Increased efficiency since hybrid heat pump solutions are energy efficient, requiring less electricity use and less overall energy consumption while still improving heating performance.
- Design flexibility for architects and owners considering additions of on-site generation from solar or wind when possible. On-site generation is more efficient since it reduces or eliminates the transmission losses found in the grid. The size of the solar system may or may not be limited by the site or the available access to the sun, meaning a supplemental or hybrid system using propane may make the most sense.