Improving Energy Efficiency in Single-Ply Roofing
Learning Objectives:
- Demonstrate how single-ply roof systems meet the requirements for cool roof building codes and achieve improved energy efficiency.
- Analyze and evaluate options to enhance the performance of a single-ply roof.
- Specify different types of roof membranes, coverboards, attachment, insulation, and vapor barriers in single-ply roof systems to achieve performance goals.
- Define and utilize best practices to assist in designing single-ply roof systems.
Credits:
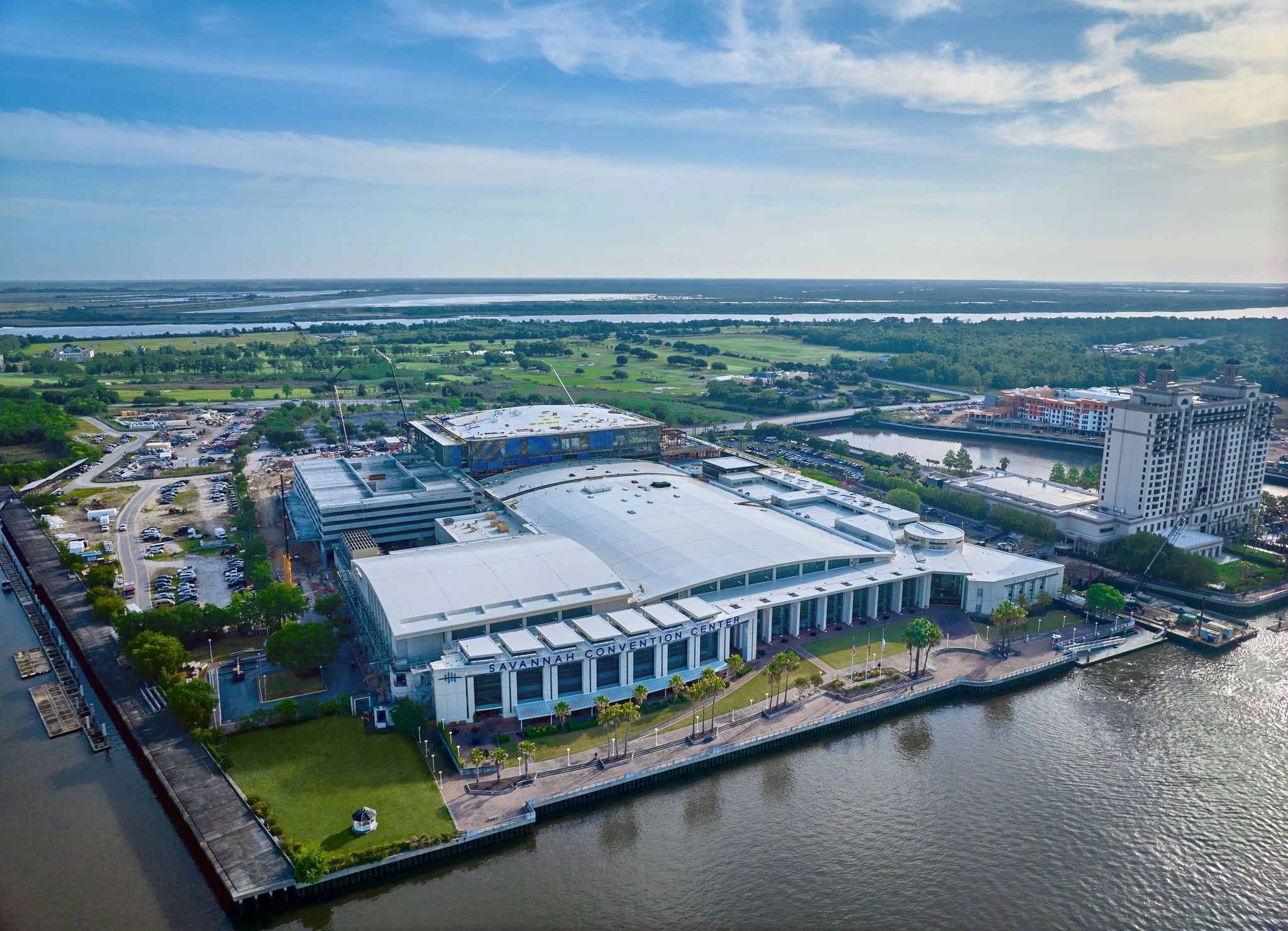
All Images Courtesy of GAF I Siplast
Sustainability at the Top: The Cool Roof and Energy Implications
When considering a single-ply roof system, building codes can offer an excellent starting point. However, code provides the minimum requirements for construction. Code represent a baseline for design and have few regulatory provisions that focus on resilience. Adding practices targeting energy efficiency and resilience can substantially reduce environmental impacts and improve existing unsustainable design, construction, and operational practices.
Designers can, and often should, design beyond the minimum code requirements to improve the durability and longevity of their roofs. Standard code requirements come from the locally adopted versions of the International Building Code® (IBC) and the International Energy Conservation Code® (IECC) and provide either performance or prescriptive requirements. The code also references specific key standards from documents issued by organizations including ASTM International (ASTM), ASHRAE (The American Society of Heating, Refrigerating and Air-Conditioning Engineers), and The American Society of Civil Engineers (ASCE).
Updated codes have increased the amount of insulation as well as air tightness, and more recently address cool roofing, all of which affects the energy efficiency of a building. Single-ply roofing systems offer many opportunities to contribute to the energy efficiency and resiliency goals of a building when the design includes careful consideration of reflectivity, insulation and airtightness. Understanding the role that each of the assembly components plays in the overall roof design and installation is key to ensuring that the roof assembly will be both durable and sustainable.
Building Code Prescriptive Requirements
Chapter 15 of the IBC provides minimum requirements for the design and construction of roof assemblies and rooftop structures. The chapter addresses Roof Drainage (1502), Weather Protection (1503); Requirements for Roof Coverings (1507); Flashing (1503.2); Coping (1503.3); Wind Resistance of Roofs (1504.1, ASCE 7); and Edge Securement, Low-slope Roofs (1504.5). These include requirements for emergency overflow drainage, gutter securement, and the ability of roofs to withstand wind events, foot traffic, and fires. Roof coverings must be designed and installed in accordance with this code and the manufacturer’s approved instructions. Code considerations must be taken into account during roofing materials selection and installation.
Energy Code – Commercial Requirements
The IECC recognizes the roof’s role in energy performance. Energy codes apply to roofs because the R-value established in the roof assembly and air barrier requirements enable containment of conditioned air inside the building. The roof's contribution towards maintaining the enclosure and keeping the interior environment separate from the exterior means that heating, ventilation, and air conditioning (HVAC) systems do not have to work as hard, resulting in reduced energy consumption.
Under IBC Chapter 13, Energy Efficiency, “Buildings shall be designed and constructed in accordance with the International Energy Conservation Code - 1301.1.1.” Designs can comply with either the requirements of ANSI/ASHRAE/IESNA 90.1 or the requirements of the IECC Commercial Provisions, which apply to all buildings except for residential buildings 3 stories or less in height.
The version of the Code is adopted at the state level; the most recent version is dated 2021, where significant updates were made in regards to the air barrier requirements. Since 2010, the IECC states that a continuous air barrier must be provided throughout the building thermal envelope (2021 IECC §C402.5.1. Air Barriers). The air barrier is permitted to be located on the inside or outside of the building envelope, located within the assemblies composing the envelope, or any combination thereof. The air barrier selected needs to comply with Sections C402.5.1.1 for materials and C402.5.1.2 for assemblies. This section is important as the roof air barrier, whether a dedicated air barrier or the roofing membrane as the air barrier, is required to be continuous across the roof as well as continuously transitioned to the exterior wall air barrier. This requirement is critical to understand when detailing the roof at both roof penetrations (discontinuities) and at the roof to exterior wall interface.
The energy code additionally sets requirements for thermal performance, including a minimum R-value for the roof. This R-value is based on climate zone and geographic location (IECC C301.1 and 90.1 Annex 1). It should be noted that the R-values in the code are denoted as minimum R-values. Owners selecting to achieve higher energy savings, or specialty buildings such as cold storage, frequently need more insulation than required by the code.
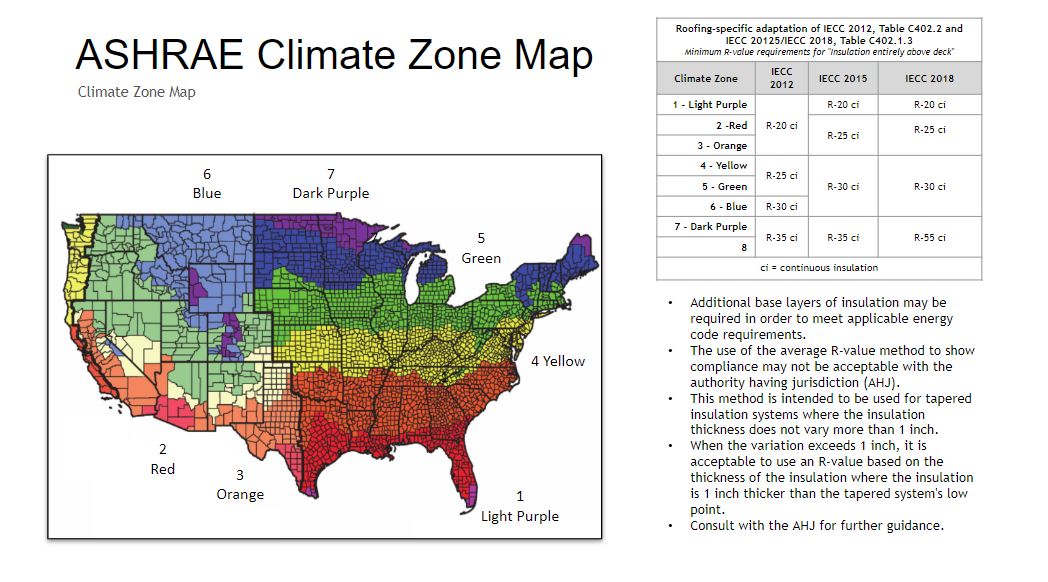
IECC Map with minimum R-value requirements.
Guidelines for installation, as provided by the codes, are also critical to achieving predicted energy efficiency. Starting in 2018, the IECC Section C402.2.1 requires two layers of insulation at the roof and provides that this installation should be staggered and offset during installation, to prevent airflow between the joints.
Cool Roofs 101
Reflective roof membranes, otherwise known as cool roofs, account for greater than 50 percent of roof surfaces installed on low-slope commercial buildings each year, and an even greater percentage are installed in the southern half of the United States. Such reflective roofs have the potential for decreasing cooling energy consumption by lowering roof temperatures (Freund et al. 2006; Ennis and Desjarlais 2009; Gaffin et al. 2010; Graveline 2013).
As defined by the Cool Roof Rating Council®, a cool roof is one “that strongly reflects sunlight (solar energy) and also cools itself by efficiently emitting any heat that was absorbed.” A cool roof does not need to be white. There are many "cool color" products which use darker-colored pigments that are highly reflective in the near infrared (non-visible) portion of the solar spectrum.
The two basic characteristics that determine the "coolness" of a roof are solar reflectance and thermal emittance. Both properties are measured on a scale from 0 to 1, where 1 is 100 percent reflective or emissive.
There is a definitive terminology associated with cool roofing. These terms include emissivity, albedo, reflectivity, and SRI.
Emissivity: Emissivity is defined as the ratio of the energy radiated from a material's surface to that radiated from a perfect emitter, known as a blackbody, at the same temperature and wavelength and under the same viewing conditions. It is a dimensionless number between 0 (for a perfect reflector) and 1 (for a perfect emitter). Simply put, the emissivity of the surface of a material is its effectiveness in emitting energy as thermal radiation or heat. Most roof membranes have an emissivity in the range of 0.85-0.95 and this is not dependent on the color.
Albedo: Albedo is defined as the ratio of the diffuse reflection of solar radiation out of the total solar radiation. It is measured on a scale from 0, corresponding to a black body that absorbs all incident radiation, to 1, corresponding to a body that reflects all incident radiation. It is commonly used in climate science as a measure of the reflectance of solar energy from the earth’s surface. Albedo is the potential for a surface to reflect sunlight. Albedo is different from emissivity in that albedo involves the radiation reflected from a surface, whereas emissivity involves the radiation emitted from a surface.
Reflectivity: Reflectivity is defined as the property of a material to reflect the light or radiation. It is a measurement of reflectance irrespective of the thickness of a material. For most reasonably thick opaque materials, reflection occurs within the first few nanometers of the surface and any light transmitted more than about 100nm into the material is quickly absorbed. Darker membranes like black EPDM have a reflectivity around 0.05, while white TPO and PVC have a reflectivity of 0.8 or higher.
SRI: The Solar Reflectance Index (SRI) is a calculated value using reflectivity and emissivity values. It is an indicator of the ability of a roof surface to return solar energy to the atmosphere. Roofing material surfaces with a higher SRI will be cooler than surfaces with a lower SRI under the same solar energy exposure, especially on a sunny day. SRI values for most materials fall between 0 and 100, although values above that range are possible. Absorptive membranes like black EPDM typically have an SRI less than 10, while White TPO and PVC membranes typically have an SRI above 80. Gray colored roofs are typically lower reflectance with an SRI around 50 and Tan is often mid-reflective with an SRI around 70. There are also specially formulated “energy” colors that are highly reflective without being white.
Many building codes reference SRI or 3-year aged SRI requirements when defining a “cool” roof. The most common of these are the California Energy Commission Title 24, the U.S. Green Building Council’s Leadership in Energy and Environmental Design® (LEED), and ASHRAE 90.1 and 189.1. The Cool Roof Rating Council® (CRRC) offers an online database to look up information on products and set desired inputs. This tool is available at https://coolroofs.org/directory/roof.
Best Practices: Building Science Principles and their Contribution to Roof Performance
How does a roof enhance the performance and efficiency of a building? It can bolster the performance by tackling three tasks: controlling for bulk water concerns, mitigating air and vapor movement, and improving thermal efficiency.
Proper design and installation of a low slope roof is important to meet both performance and building code requirements. As more materials and additional code requirements are added to enclosures, including air tightness, it is important to recognize when materials and assemblies need to adapt in order to accommodate these changes. Most single-ply membranes are Class I vapor retarders, which mitigate moisture migration when they are detailed properly, however, they can also trap moisture within the roof system.
As energy performance is improved in building enclosures, moisture risks can increase from decreased heat flow across the assemblies. Historical roof systems consisted of dark surfaces with little or no insulation. While this did not promote energy efficiency, it allowed for condensation that may have occurred within the assembly to dry into the interior of a building. Today’s roofs often consist of reflective surfaces that reflect heat away from the surface, which do not equally provide the heat that allows for roofs to ‘dry down’ if condensation occurs. Unintended and uncontrolled air movement, vapor movement, and discontinuous control layers can inadvertently bring and trap moisture into the roofing system. Modern day roof materials and their interactions across the roofing assembly, as well as at the roof to wall interfaces, are important to understand so that moisture is adequately controlled.
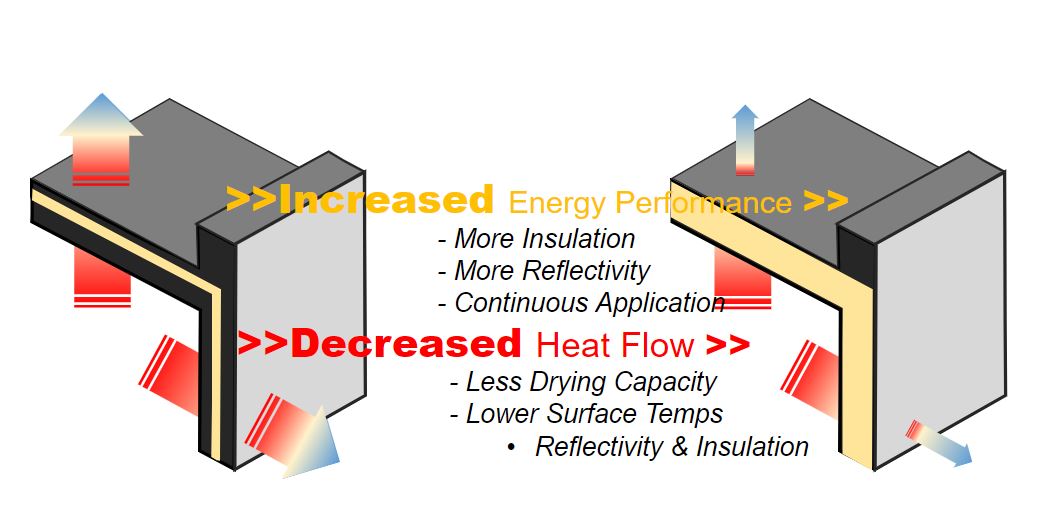
Mitigating Moisture Concerns - Bulk Water
The first reason for installing roofs is to keep water out of buildings. While there are many types of roof assemblies available, there are three main considerations for managing water intrusion when designing or selecting a roof. Controlling bulk water, such as water from rain or snow, can be accomplished by considering roof slope, membrane seams, and durability such as mitigating damage from storms.
The impacts from moisture intrusion are many, including roof leaks causing headaches to occupants below. Leaks from the roof cause costly repairs, but they also can deteriorate the roof assembly and roof structure, and lessen its performance, including reducing R-values in the insulation. Wet insulation can have a reduced R-value to almost zero when saturated. Water, once it enters into the building, can not only damage interior finishes, but prolonged water infiltration can facilitate mold growth. Mold growth will ultimately affect indoor air quality as it may enter the airspace of the building.