The Climate-Positive Benefits of Concrete in Outdoor Architecture
Recent innovations in the composition, manufacture, and use of this resilient material
Continuing Education
Use the following learning objectives to focus your study while reading this month’s Continuing Education article.
Learning Objectives - After reading this article, you will be able to:
- Discuss the need to reduce carbon and other greenhouse gases in the atmosphere.
- Describe the manufacture of concrete, its use worldwide, and technological advances.
- Identify climate-positive outdoor design.
- Define the impact of material choices in outdoor architecture.
- Describe climate-positive projects where concrete plays a critical role.
Outdoor architecture has a vital role to play in the coming years to achieve net zero and carbon negative in the built environment, and it requires a more in-depth consideration of durable materials and their proper use. The resilience of concrete in outdoor design is well known. Still, more understanding is needed about modern concrete, the savvy use of this impressive material, and its actual impact on the environment. Specifying permeable pavers is an excellent example of an intelligent design strategy that decreases material use but increases environmental benefits. This course takes a deep dive into the use of concrete in outdoor architecture to benefit people and the planet.
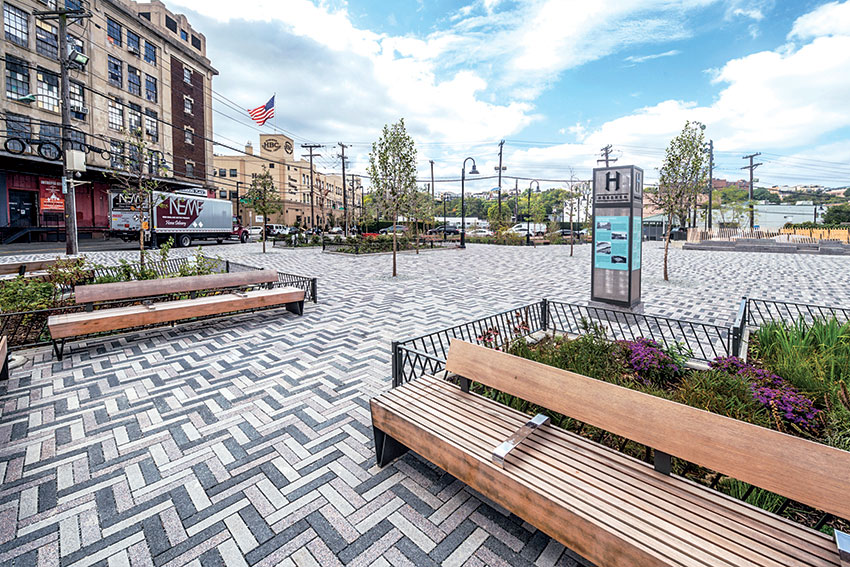
Photo courtesy of Unilock
Aesthetically pleasing and durable, permeable pavers help prevent flooding in parks, plazas, and other outdoor spaces.
HOW OUTDOOR ARCHITECTURE HELPS MEET SUSTAINABILITY GOALS
Within the design/build industry, architects and designers have long taken the lead toward creating a sustainable future. That is true for the creation of indoor spaces and outdoor spaces alike. “As a firm, we strive to leave our world better than we found it,” says Hana Ishikawa, design principal with Site Design in Chicago. Toward that goal, the firm has designed several pocket parks with permeable surfaces that help collect rainwater to prevent street flooding and also fill underground aquifers. “As the topic of global warming becomes an increasingly important discussion,” Ishikawa adds, “permeability in our hard walking surfaces becomes increasingly important.”
The New York City Department of Parks & Recreation (NYC Parks) has the same focus.
“Planning for the long-term resiliency of our open spaces is a primary objective of NYC Parks,” says NYC Parks Press Officer Gregg McQueen. “The use of permeable pavers has proved to be an effective means of managing stormwater, reducing the environmental impact of paved spaces and helping Parks create a more sustainable and resilient city.”
Resiliency in Hoboken, New Jersey
A prime model of beneficial outdoor architecture is Southwest Resiliency Park, located in a chronically flooded, formerly industrial, lowland district in Hoboken, New Jersey, along the Hudson River. Today there lies a jewel of a one-acre pocket park that is as functional below ground as fantastical above ground. The popular park, built in 2017, features a dog run, restroom, pop-up market space, amphitheater, open lawn, and playground space to promote year-round social activity. This is evidenced by kids running and playing, people reading, visiting each other, and dogs playing.
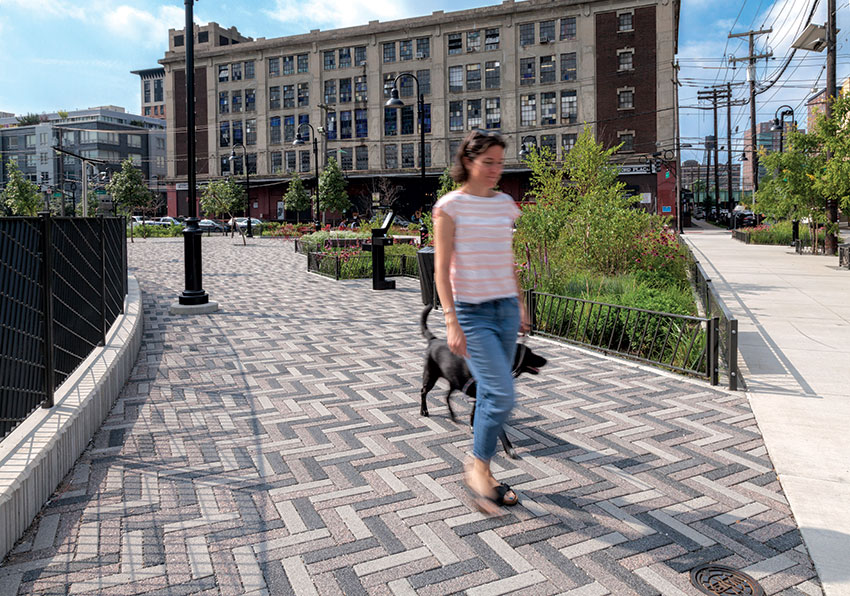
Photo courtesy of Unilock
The durability of permeable pavers helped achieve the designers’ goals for the Southwest Resiliency Park in Hoboken, New Jersey.
The heart of the space is an open area paved with eye-catching open-jointed concrete pavers, also known as permeable pavers, which give the site a high-quality aesthetic that is both sophisticated and lively. But the real beauty of the park is its role in Hoboken’s water management strategy to delay, store, and discharge stormwater that, during extreme rain events, has overwhelmed the combined sewer system and flooded low-lying areas in the neighborhood.
The various layers of the system—seen and unseen—include the open-jointed permeable pavers, tree cells with aerated soil to collect water, rain gardens, and street bioswales. These systems lead to the subsurface detention area that can store up to 200,000 gallons of water from significant storm events. This park sets a new standard for integrating urban public space with storm protection.
It’s hard to see how this resilience-focused project could have been accomplished without one of the most resilient materials on Earth—concrete. Permeable concrete pavers to help prevent flooding have been used in parking lots where giant yellow buses drop off and pick up school kids, as well as in shipping ports where heavy tractor-trailers roll up to pick up loads of cargo. Even when concrete's strength and versatility are acknowledged, the question remains: What about carbon emissions?
“For designers working on projects with specific carbon targets, the evaluation and material selection process is complex,” says Elaine Willis, Director of Sustainability at Unilock. “It isn’t as simple as selecting products with low or zero embodied carbon, but understanding how the product will work in situ to create a system that provides multiple environmental benefits, including reducing and sequestering embodied carbon from the environment.” Willis continues: “We are seeing innovations across the whole spectrum of the concrete industry, from alternate cementitious materials to efficiencies in the manufacturing processes, in our facilities, and in our suppliers. It will be the culmination of all efforts that will get us to net zero.” {{question1}}
CONCRETE AND CARBON EMISSIONS
When designing for a sustainable future, the goal of net-zero carbon emissions is typically front and center as a concern. “Working toward net-zero carbon emissions requires designers to place a magnifying glass over all materials and operations,” says landscape architect Misa Inoue, PLA, ASLA, and project designer Jason Vogel, LEED AP BD+C, both with Ross Barney Architects. They worked on the McDonald’s Chicago Flagship Restaurant, which will be discussed later in the course.
Much has been written about the amount of concrete used worldwide and its associated carbon emissions. But these statistics need more context. Rarely is concrete's incomparable strength and durability factored into the discussion. Many would be surprised to learn that carbon emissions associated with concrete are less than even educated people realize.
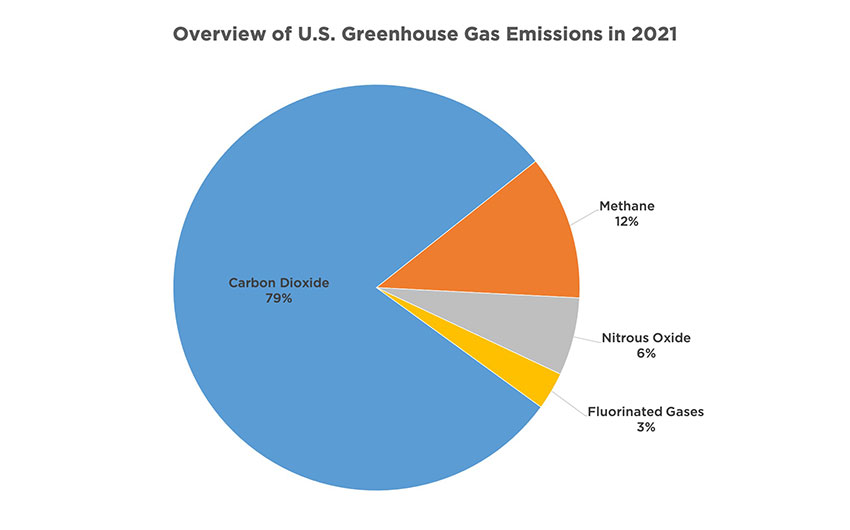
Image courtesy of Unilock; Source: “Overview of Greenhouse Gases”
The comparative percentage of gases in the atmosphere.
The Need to Reduce Carbon and Other Greenhouse Gases in the Atmosphere
The Earth’s atmosphere relies on a balance of greenhouse gases (GHG) to reflect heat from the sun, allowing some heat to escape and retaining what flora and fauna (including humans) need for a comfortable, habitable planet. The past century has seen advances in modern society, such as motorized vehicles, heating and cooling systems for buildings, and manufacturing. All of these traditionally use fossil fuels. Compounded by deforestation and urban development, human activity has created an imbalance in the GHG, primary carbon dioxide (CO2). This has resulted in a global temperature rise and significant weather events.
“Embodied carbon” is the globally chosen value to measure the movement toward a net- zero environment. The definition of embodied carbon is the carbon dioxide equivalent relative to the global warming potential (GWP). Therefore, embodied carbon and GWP are often used interchangeably. For structural materials, embodied carbon is linked to the extraction of the raw material, manufacturing, transportation, construction, maintenance during service life, demolition, and end of life. This measurement allows designers to manage choices for materials. Hence environmental product declarations (EPDs) are created for products, leading to more informed product and design decisions. However, a simple number only tells part of the story.
Other Gases in the Earth’s Atmosphere
Before discussing the building materials and environments, it is essential to understand how carbon dioxide stacks up against the other elements that make up the atmosphere. There is more CO2 in the atmosphere than other gases, but those other gases have a much more significant effect in smaller quantities.
Methane: According to the Environmental Protection Agency1, one pound of methane has a 25 times greater negative effect over 100 years than one pound of CO2. The typical sources of methane in the environment are from agriculture, natural gas, petroleum systems, and landfills.
Nitrous oxide: One pound of nitrous oxide has the same global warming effect as 22 pounds of carbon dioxide in the atmosphere. The majority of that is from agricultural soil management.
Fluorinated gases: Fluorinated gases (gases that were created to replace chlorofluorocarbons) and hydrochlorofluorocarbons (which are old refrigerants used in building and vehicular air conditioning systems) have a high global warming potential and stay in the atmosphere for thousands of years. These are released during manufacturing processes, leaks, servicing, and equipment disposal. A new subset of HFCs is being developed with short atmospheric lifetimes and lower GWPs. {{question2}}
Embodied Carbon vs. Operational Carbon
The built world accounts for 37 percent of global emissions. Building materials and construction equals 9 percent, including renovations and new builds, while 28 percent is caused by building operations. The most significant source of human-generated CO2 emissions is fossil fuel power plants, at 36 percent in the chart. In the U.S., passenger vehicles and light trucks generate another 20 percent, while this value is 22 percent globally.
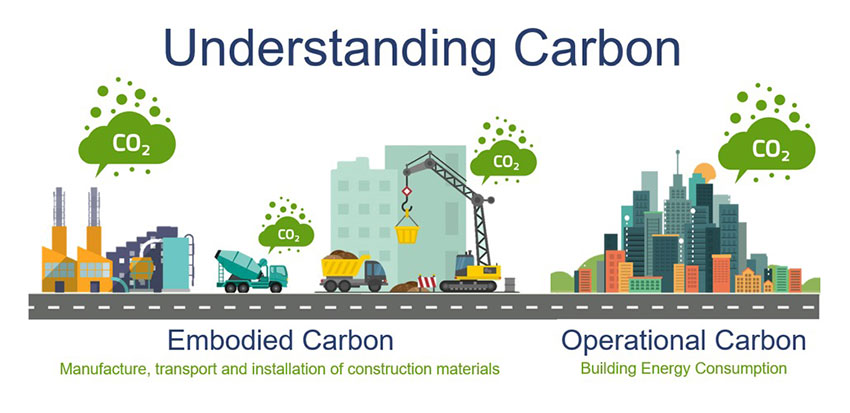
Image courtesy of Stacy Smedley, SKANSKA/CLF
There is a difference between embodied carbon and operational carbon.
Globally, cement accounts for 7 percent of emissions, primarily due to the intensive process of extracting and creating this material with the characteristics required by the built environment. Though well below the emissions of agriculture, power, and transportation, this quantity is not insignificant. It is also important to note that cement manufacturing accounts for less than 1.5 percent of all U.S. human-generated CO2, according to the Portland Cement Association.2
Over the last century, the cement industry has reduced carbon dioxide emissions per ton, but production is growing substantially worldwide due to economic growth, notably in developing countries. Compounding this issue is the shorter life cycle of concrete buildings in China, increasing the need for cement. In North America, the lifecycle of a building averages 60 years. However, in China, the average lifecycle of a building averages only 35 years. According to the Journal of Cleaner Production, “China has about 60 billion m2 of existing buildings, with approximately 2 billion m2 newly constructed each year. This accounts for half of all new buildings globally.”3
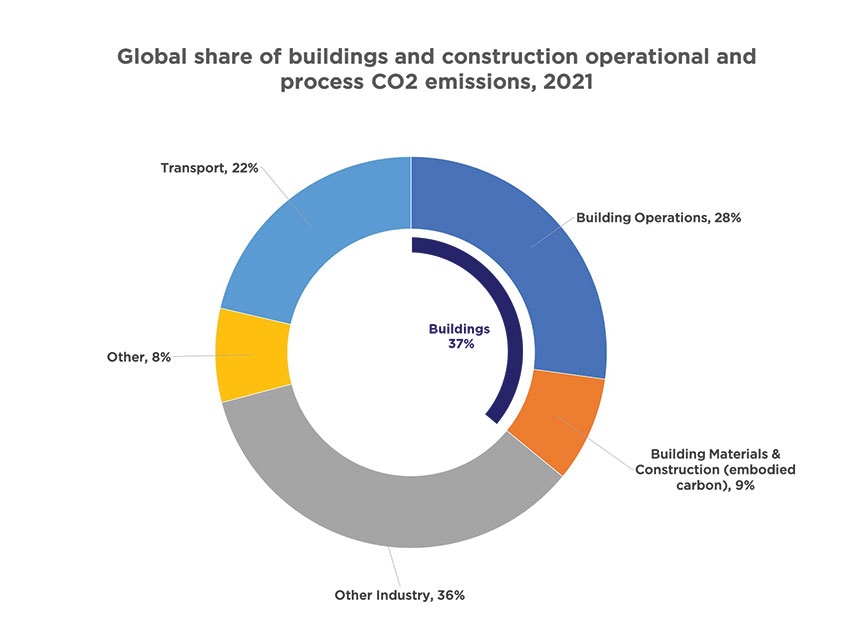
Annual global emissions by source.
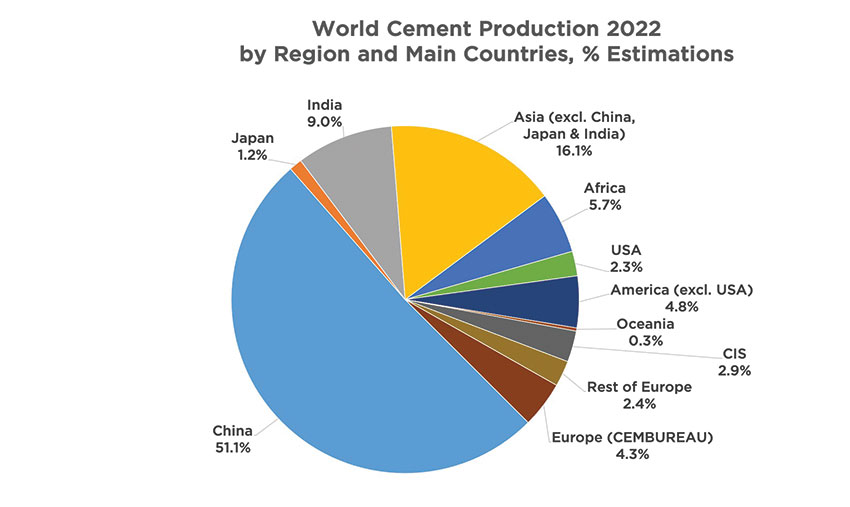
Image courtesy of Unilock. Source: World Cement Production 2022
China’s use of concrete far exceeds that of other countries.
{{question3}}
{{question4}}
THE MANUFACTURE OF CONCRETE, ITS USE WORLDWIDE, AND TECHNOLOGICAL ADVANCES
Cement or cementing materials date back to Roman mortars and concretes. But it wasn’t until the 1820s that Portland Cement was created and patented in England. Today’s concrete includes many types: normal strength, reinforced, pre-stressed, poured in place, precast, lightweight, high-density, and more. But the general manufacturing process is the same. Raw materials such as limestone silica, alumina, and gypsum are extracted from the earth. They are then heated at extremely high temperatures to create a chemical reaction called calcination. This reaction produces the clinker, which is mixed and ground to make cement. Cement is then taken to the concrete mixing facility (this could be the same site or a different location) and mixed with water, air, and aggregates to make the concrete. It is then transported to the location of use to create the built environment, either in a wet or cured format. To close the loop on its life cycle is the end of life, which for some concrete products can include reuse or recycling as an option.
The most significant CO2 impact happens during the creation of the cement. During cement manufacturing, emissions related to fuel use (to create the heat) account for 40 percent of the material’s total CO2 creation. The remaining 60 percent comes from quarrying, transport, cooling, and mixing, but mostly from process emissions which include the chemical reaction called calcination required to make cement clinker. Almost a pound of CO2 is emitted for every pound of cement. However, cement is only one ingredient in concrete. Some 85 to 90 percent of concrete is made up of other ingredients. That means 10 to 15 percent of the mixture is cement; the rest is water, aggregates, admixtures, and air.
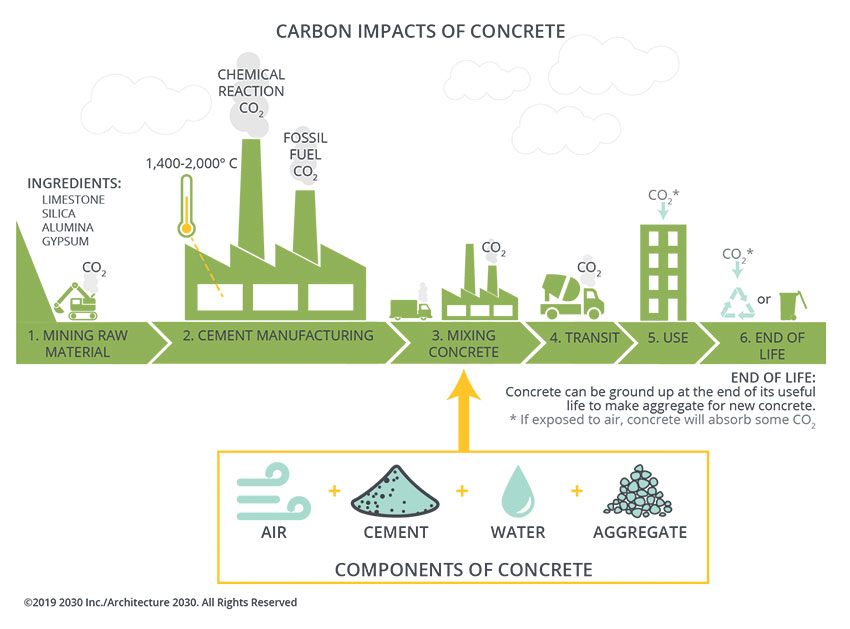
Image courtesy of ©2019 2030 Inc./Architecture 2030. All Rights Reserved.
There is a difference between embodied carbon and operational carbon.
Concrete Alternatives
In the early 1990s, concrete manufacturers started to replace some of the cement in the mix with supplementary cementitious materials (SCM). Today, some manufacturers continue actively working to reduce the cement content in concrete, up to 55 percent in some cases. Manufacturers that don’t need freeze-thaw test ratings can replace even more cement. However, performance must be factored in. More cement is required to create concrete with high compressive strength, which means an excellent freeze-thaw rating, essential for durability in colder climates.
Potential replacements include:
- Pozzolans: Fly ash, volcanic ash, glass, and rice husk, to name a few, combine with calcium hydroxide to bind together.
- Calcined clay or metakaolin: This is technically a pozzolan but more reactive than some others and is readily available.
- Bio concrete: These are natural materials such as microalgae or wood waste. But many of these materials are unable to create sufficient compressive strength.
- Carbon capturing material: This is used in the aggregate mix design and uses a cementitious material to bind it all together.
Developing materials that meet required compressive strengths, flexibility, and freeze-thaw standards remains difficult. .
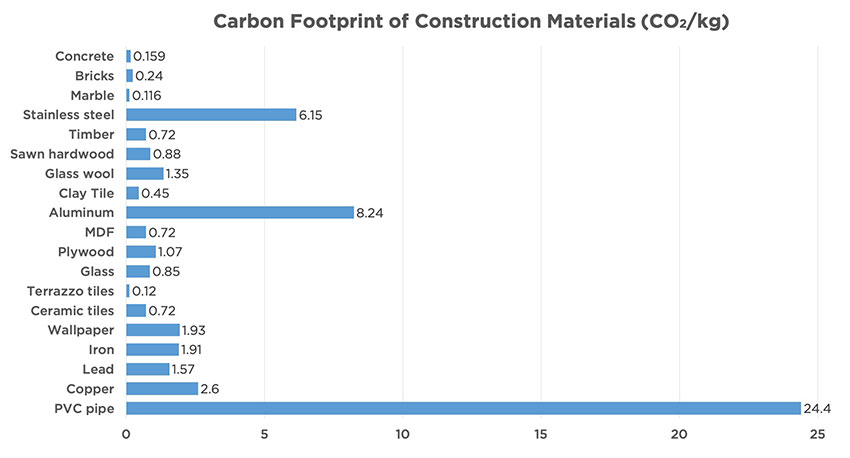
Image courtesy of Unilock; source: Carbon Footprint of Building Materials (Green Building Calculator)
Graph #1: Comparison of the carbon footprint of construction materials.
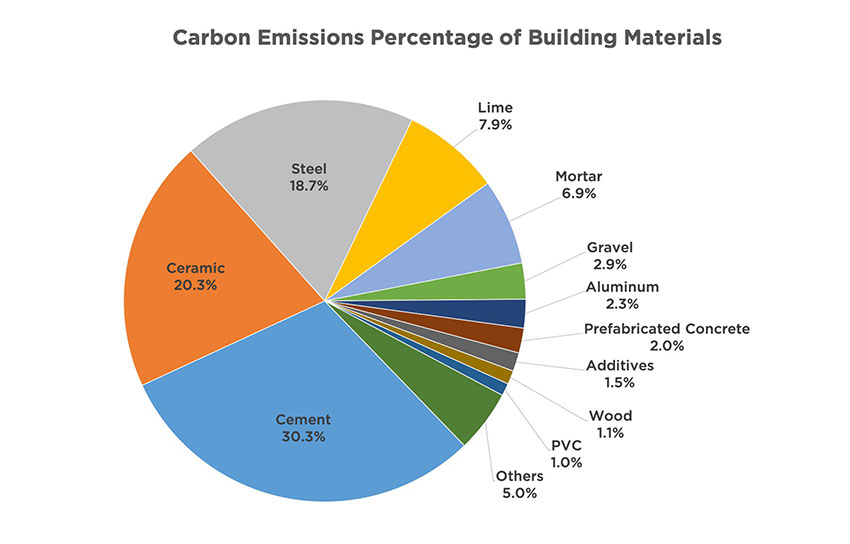
Image courtesy of Unilock; source: Carbon Footprint of Building Materials (Green Building Calculator)
Graph #2: Carbon emissions by percentage of building materials
Getting Concrete to Net-Zero Emissions
Within cement and concrete associations across the globe, many are working on scalable solutions to get to net-zero carbon emissions. This is not a simple ingredient fix but a combination of solutions across the manufacturing process. This includes:
- Efficiency in design and construction
- Efficiency in concrete production
- Savings in cement and binders
- Savings in clinker production
- Carbon capture and storage
- Decarbonization of electricity
Recarbonization
Recarbonization means carbon that is reabsorbed or soaked up. This is referred to as carbon uptake or a carbon sink. To understand how this happens, it’s important to know that the chemical reaction called hydration takes place in concrete. This is a reaction between cement (or substitutes) and liquid, which is what makes it harden. And this process continues well beyond the 28 days when concrete typically reaches peak strength.
Recarbonization takes place when concrete is exposed to the environment. Carbon dioxide penetrates the concrete capillary pore network and is dissolved when it reacts with the calcium ions and other alkaline species produced by the cement paste. Studies have also shown that the recarbonization rate increases with some supplementary cementitious materials, such as slag.
A 2010 study included this note in its conclusion: “The net carbon dioxide emissions related to the Portland cement clinker industry (CO2 released in clinker fabrication minus CO2 uptake by concrete carbonation) should be implemented in the climatic models of the next IPCC assessment report.”4 However, at this time, the Intergovernmental Panel on Climate Change (IPCC) currently does not consider the carbon absorption value. The Massachusetts Institute of Technology is working on this, with the concrete industry awaiting the results.
As more buildings are made from cement, the uptake rate increases as these structures age or are replaced, particularly with the deconstruction or crushing of concrete which increases the uptake rate significantly. Of course, there would also be an offset for the process or energy required in recycling the concrete to be accounted for. {{question6}}
Carbon Footprint of Building Materials
Embodied carbon is used to measure the GWP of building materials, which is then added to the operational carbon, meaning the resources and energy that the built environment will consume over its lifespan, to come up with the Total Whole Life Carbon value. Graph #1 (left, upper) above shows the carbon footprint of various materials used in construction. The measurement is by weight, which is how all embodied carbon materials are measured. Plastics and metals have some of the highest footprints, and concrete comparatively the lowest, but obviously, there is more weight to concrete and stone than PVC pipe.
Additionally, how much of each material used in building construction needs to be factored in. Cement is usually responsible for the highest portion of the built footprint, not just a building but infrastructure, and cement is used extensively. Graph #2 (left, lower) shows prefabricated concrete with a value of 2 percent. One can assume they are referring to a material such as pavers, but it could also include other precast features. So, there is an extensive range of products under the cement category. For buildings, designers can use specific design techniques that can decrease operational consumption or even build in offsets (such as adding solar power) to have all new buildings be net-zero carbon by 2030, and all buildings be net-zero carbon by 2050. This goal is set by the World Green Building Council and adopted by the Architecture 2030 Challenge.
WHAT IS CLIMATE-POSITIVE OUTDOOR DESIGN?
Climate Positive Design (CPD) was founded in 2019 as part of a research initiative supported initially by the Landscape Architecture Foundation (LAF) Fellowship for Innovation and Leadership. Climate Positive Design is not just about the singular product selections but how the project can be designed to balance selections with embodied carbon with features that will actively work to sequester carbon. This includes increasing planting, using suitable soil mixes, increasing native and species varieties on site, such as urban plazas, pocket parks and roof decks, replacing or improving coastal wetlands, and creating multifunctional green roofs. {{question7}}
Today’s sprawling urban environments create more emissions, increase local temperatures, decrease run-off opportunities, increase chances for flooding, and mix run-off with outdated combined sewer systems compounding pollutants in waterways. The need for carbon sinks, green environments, and water runoff management has never been greater. One of the most natural carbon sinks is soil. Combined with plant materials and other easy design details can address the above. Globally, landscape architects and architects have made climate action commitments with tangible goals and objectives with specific timelines. In 2018, the American Society of Landscape Architects (ASLA) joined the America Is All In movement, a national coalition of 4,100 states, cities, companies, and organizations that, ALSA says, “remain committed to achieving US greenhouse gas emission reduction targets as part of the 2015 Paris climate agreement. ASLA was one of the first organizations representing the built environment community to join the coalition.”
More recently, the ASLA Board of Trustees ratified the International Federation of Landscape Architects (IFLA) Climate Action Commitment, joining a coalition of 70,000 landscape architects in 77 countries in committing to limiting planetary warming to 1.5°C (2.7 °F). This is the largest coalition of landscape architecture professionals, the ASLA states, ever assembled to advance climate action. Also, ASLA became an official signatory of the Architecture 2030 1.5°C COP26 Communiqué. ASLA joined with Architecture 2030 to “call for all sovereign governments to reduce greenhouse gas emissions by 65 percent by 2030 and achieve zero emissions by 2040, which would accelerate the current timeline to achieve emission reductions outlined in the Paris Climate Accord by a decade.”
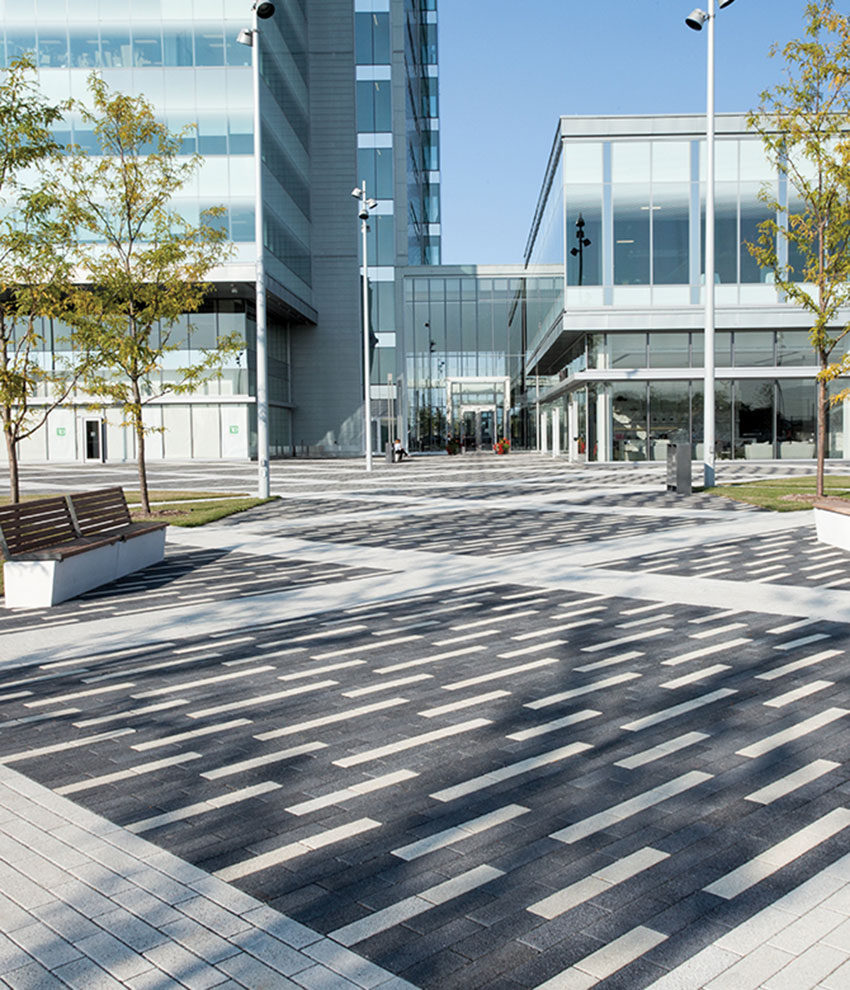
Photo courtesy of Unilock
This 10-acre park with artfully designed pavers acts as the backbone of a transit-oriented development while linking to smaller, more intimate neighborhood parks.
Walkable Communities
There is a much larger scope to consider than simply the embodied carbon value of an individual product. A holistic approach is required to look at the full scope of design and how it will affect everyday lives. The concept of walkable communities is an excellent example of this. If we continue to create more walkable communities, we can reduce the need for singular transportation. That is significant as transportation makes up 29 percent of emissions, with 58 percent of that value from passenger vehicles. Improved transit systems, biking routes, and safe pedestrian options enhance walkability. This not only has positive health improvements but will save citizens money. That money can be spent in the local economy. This tactic includes mixed-use developments and higher-density developments close to transit access.
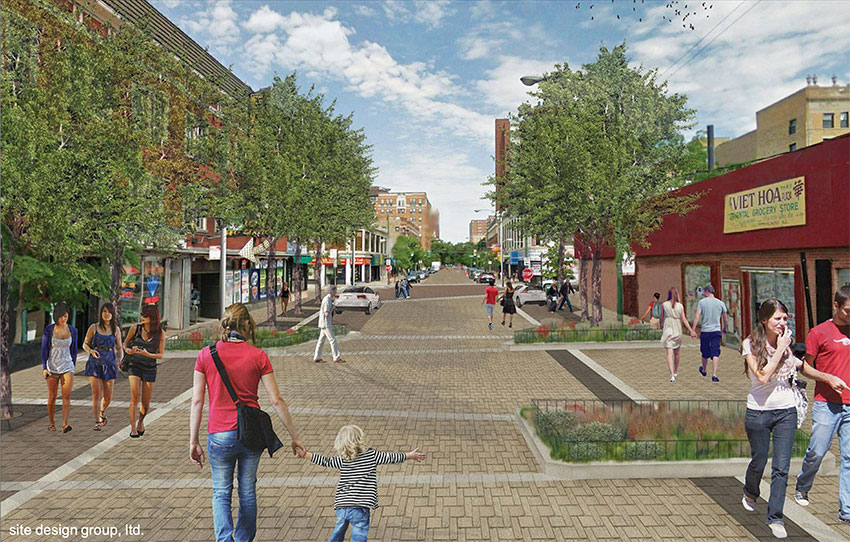
Image courtesy of site design group, ltd.
This “shared street” in Chicago is friendly to pedestrians and bicyclists as well as slow-moving vehicles.
Vaughan Metropolitan Centre
An example of transit-oriented development is the Vaughan Metropolitan Centre just north of Toronto, Ontario. With plans solidified for an expanded bus and subway network, a master plan was created to transform this area into a dynamic urban hub.
The 60-hectare (150-acre) development connects to the Greater Toronto area, and the 10-acre park acts as the backbone while linking to smaller, more intimate neighborhood parks. This creates a vibrant downtown core for Vaughan that thoughtfully integrates mobility and lifestyle while delivering all amenities and necessities for young and not-so-young.
Complete Streets
Complete streets have been recognized as a critical component of walkable communities and a natural complement to sustainability efforts. They promote mobility, community, and many elements of street design, construction, and operation that can improve environmental sustainability. By narrowing traffic lanes, adding bike lanes, and increasing walkways, mobility and accessibility of the space improve while also creating an opportunity to make it programmable for the community.
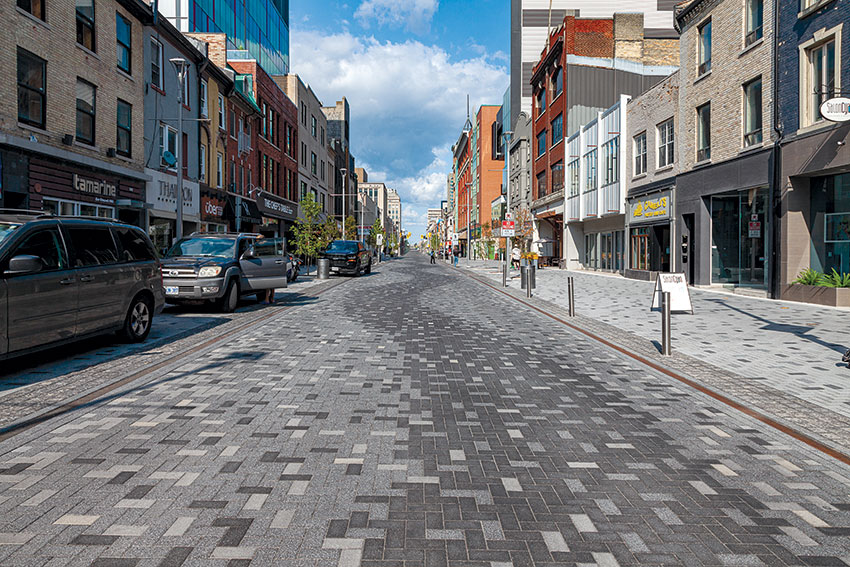
Photo courtesy of Unilock
The pavers used for Dundas Place, in London, Ontario, mimic the flow of the nearby Thames River.
Dundas Place in London, Ontario, was reimagined with collaboration from the city residents and business owners to create a multifunctional, multi-programmable space that realigns the pedestrian realm to be more prominent than the vehicular realm.
The materials chosen make it more tactile and create an atmosphere celebrating its history and cultural purpose. The design mimics the nearby Thames River, and the road leads there. The flexible corridor, which includes concrete pavers, offers many experiences along its length, exuding innovation, vibrancy, creativity, and entrepreneurialism, making this section the civic heart and a favorite place of Londoners.
Reduce Nonpermeable Surfaces
Features such as permeable pavers, bioswales, planters, gardens, and trees reduce stormwater and flooding issues, increase the quality and purity of groundwater, and reduce the heat-island effect. At the same time, these systems sequester carbon, whether in the concrete or planting systems.
Argyle Street, Chicago’s first shared street, (a roadway designed for slow travel speeds where pedestrians, cyclists, and motorists all share the right of way) aims to increase safety and create a sense of place for the community to shop and gather, while also capturing and infiltrating the majority of the stormwater. Some 35,000 square feet of permeable pavers delineate pedestrian, parking, and shared space. Real-time sensors gather data about water management capabilities that will help inform future projects.
The redesign achieved a 31 percent reduction in impervious surface area, diverting 89 percent of stormwater from the grey infrastructure system. Retailers have also reported improved shopping and dining environments and a more attractive, programmable streetscape and outdoor setting. The project was designed by site design group, ltd. (site). {{question8}}
Permeable Paving Systems
A permeable paving system is a viable component of any green infrastructure. It improves stormwater management using detention control or volume control design options, improves local water quality, reduces flooding, and decreases the chance of heating waterways with runoff. Permeable pavers are highly aesthetic, durable, and easy to maintain.
Starting at the top, a permeable paver system includes nine layers:
- Permeable paver
- Joint aggregate
- Setting bed
- Base aggregate
- Sub-base aggregate
- Subgrade
- Edge restraint
- Underdrain
- Mechanical base stabilization
Research studies report5 that GHG emissions are 78 to 95 percent lower for permeable pavements and bio-retention basins than for conventional drainage systems.
When replacing conventional asphalt pavement, the reduction is about 46 percent. The life-cycle cost of permeable pavements can be up to 17 percent lower, and potentially more, depending on the design, and maintenance costs are also lower.6,7 The permeable paver options ensure design flexibility and creativity for many applications, including vehicular, pedestrian, and heavy-duty.
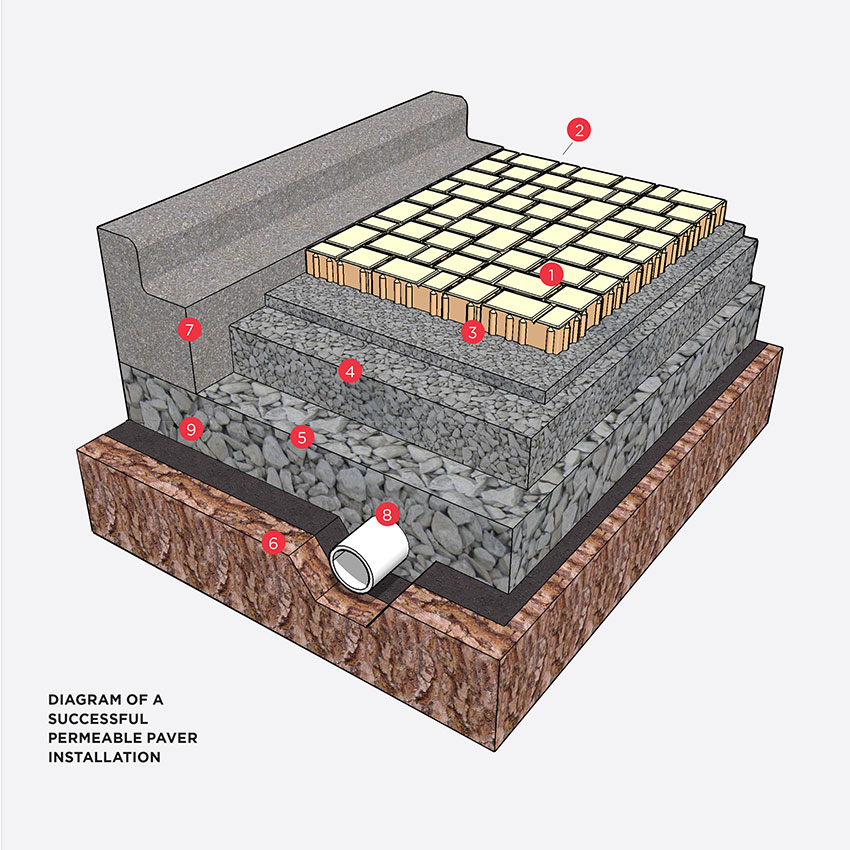
Image courtesy of Unilock
A permeable paver system includes nine layers.
Another key contributor to climate-positive design is incorporating solutions to help address the heat island effect, particularly in urban settings where a concentration of buildings, vehicles, heaters, and air conditioning units along with dark surfaces such as roadways absorb heat and increase the urban temperatures more significantly when compared to rural settings. Among the solutions of adding trees, shade structures, green roofs, and walls, creating cooler streets and pavements is essential. This is easily accomplished by selecting concrete and unit pavers with high Solar Reflectance Index (SRI) values. All of these green infrastructure elements help to reflect solar rays instead of absorbing them.
Pavers vs. Poured-in-Place
To give this more context, consider the mass value of concrete used in a hypothetical project, comparing poured-in-place (PIP) to precast pavers. Pavers offer the opportunity to save up to 60 percent of the mass of concrete volume. This is entirely dependent on the design.
In a reference project, 150mm thick PIP concrete was specified for pedestrian spaces, with a 60mm precast paver specified for the same application. As 60mm is 40 percent of 150mm, the savings is 60 percent of the mass. Now the strength of the concrete also dramatically influences the embodied carbon value. The higher the strength, the higher the value because more cement is required. Pavers are always made to meet and exceed 8000 psi, while PIP can be as low as 3000 psi. When the embodied carbon value for the material selection is correlated, there is an opportunity to reduce the embodied carbon by at least 25 percent when using precast pavers rather than PIP, with exact calculations dependent on an actual project.
Engineered Walls and Segmental Retaining Walls
When considering retaining walls, comparing cast-in-place to segmental retaining walls (SRW), the environmental consequences are design-dependent. The material volume may be about the same in some cases, whereas other designs may see a significant advantage for segmental retaining walls. A report from Brazil titled “Comparative study of retaining walls aiming to optimize carbon footprint”8 compared reinforced concrete cantilever walls to mechanically stabilized earth (MSE) with a geogrid-reinforced SRW. The CO2 emissions of the SRW were 75 percent less than PIP walls. Add to that the end-of-life scenario and the ability for SRW to be reused and reconfigured; there is a definite advantage for SRWs.
Each site needs careful evaluation for every engineered application to determine the best practice. This will depend on the soil conditions, the allowable space for the system, the aesthetic finish, and the project's environmental goals. Although it can seem counter-intuitive to use manufactured materials that seem to have a high carbon value, the amount of these materials used in the system (soils, grid, and SRW block and engineering input) can be substantially less than traditional ground engineering solutions. Additional consideration can be given to gravity-engineered walls as there is less disruption to native soils and required backfill. {{question9}}
{{question10}}
THE IMPACT OF MATERIAL CHOICES IN OUTDOOR ARCHITECTURE
Materials matter in outdoor architecture, and each must be weighed against various criteria. A material with a low-carbon-emitting score that is short-lived and needs to be replaced often may not be the best choice. Here are factors to consider.
Tools to Measure Environmental Impact
As we grapple with the complex situation in which we find ourselves amid a climate crisis, new tools emerge to measure the environmental impact of materials. The Embodied Carbon in Construction Calculator (EC3) tool compares different suppliers of a specific material. Beacon and Tally look at the LCA for a whole building. Pathfinder and Carbon Conscience were developed explicitly for landscape projects and encompass a variety of material choices. Many more tools are available, and new programs are constantly coming online.
Environmental Product Declarations
At the center of all these tools is the Environmental Product Declaration (EPD) which calculates the embodied carbon value of products for the built environment. There are four types of EPDs: 1) Industry-wide, with the least specific data; 2) Product specific, which could be manufactured at multiple facilities; 3) Supply chain specific, which is a product-specific EPD that uses supply chain-specific data in the LCA to model impacts of key processes upstream, and 4) Facility-specific EPDs. EPDs are developed according to Product Category Rules (PCR) so that all products within the same category are created similarly. Lastly, they are verified by a third party. With this data, designers, and specifiers have context for choices.
Understanding and comparing various concrete products within their unique categories is important. Again, because compressive strength is important to the integrity and durability of a product such as a unit paver, it should not be compared to a cement product created in a concrete masonry unit (CMU) category. Ensure that the Product Category Rules, ISO, and ASTM standards align between the EPDs being compared.
Recycled or Recyclable Materials
The impact of product choices is not always apparent. There may be the option to use recycled steel or plastic materials, use reclaimed or sustainably harvested materials, or reduce some materials. It’s also important to understand how the project will be deconstructed at its life’s end and what can be recycled or reused.
Materials can be optimized. For instance, specifiers can use performance-based specs, allowing cement substitutes in the mix design to explore alternatives to poured-in-place materials. The process for any reuse or recycling will also need to be considered to estimate the carbon balance of the process or energy usage and type for the recycling process. And there may also be many new types or alternative methods that will evolve in the coming months or years.
Natural and Local Materials
Using native and resilient plants allows less need for transportation to the site and less maintenance or replacement over time. Likewise, specifying concrete products manufactured closest to the project also reduces carbon emissions. Ideally, the manufacturer of the preferred product maintains multiple plants around the country.
Durable and Long-Lived Materials
While a material might seem like a low-carbon choice, the initial calculations are skewed if that product is not resilient and often needs to be replaced or repaired. Materials durable enough to last decades will ultimately cause fewer emissions.
Maintenance Matters
Understanding the maintenance requirements of a project once it is built is critical for sustainability. This could lead to incorporating features to facilitate ease of maintenance. This could include onsite composting to shore up the soil beds created. Consideration should be given to avoiding fossil fuel-based fertilizers and powered lawn equipment.
End-of-Life Issues
Understanding the end-of-life options for the projects will need to be considered by material and weighed for the advantages and disadvantages of each. This includes removal from the site, transportation, energy, and reuse or disposal.
CLIMATE-POSITIVE OUTDOOR PROJECTS WHERE CONCRETE PLAYS A CRITICAL ROLE
These park and pocket park projects illustrate how concrete plays a critical role in climate-positive outdoor projects. The insertion of pocket parks into urban centers has many environmental and health benefits for the community.
Jemmy's Dog Run, Madison Square Park, Manhattan
Located just south of Madison Square Park's West 25th Street entrance, Jemmy’s Dog Run is a year-round community park for dogs, aptly named after founding father and fourth president of the United States, James “Jemmy” Madison. The dog park is popular for locals to bring their pets to frolic, socialize, and play. The Madison Square Park Conservancy enlisted the expertise of designer SWA/Balsley to envision a new design for the dog run within this historic New York City landmark. The newly designed space complements the park’s Victorian-era charm while integrating such modern features as water-fill stations for the dogs, climbing mounds, upgraded K9 turf, and the installation of permeable pavers.
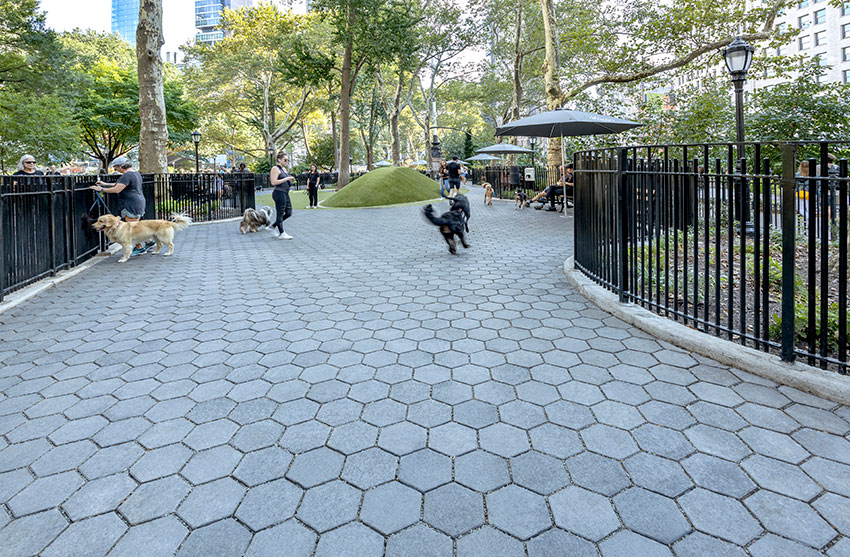
Photo courtesy of Unilock
Jemmy's Dog Run, Madison Square Park, New York City.
The park’s contemporary ambiance was elevated with hexagon pavers in a midnight sky color, delivering a streamlined and polished appearance. The decision to opt for these pavers was driven by their permeable application, allowing for greater absorption of excess rainwater into the subsoil through wider joint gaps. This design approach not only helps prevent flooding but also prevents freezing during colder months. The distinct finish has a mottled surface akin to natural granite, accentuating its appeal under sunlight's warmth. The finish also delivers a safe, slip-resistant texture for pets and pet owners.
Yolanda Garcia Park, Bronx,
Named in honor of a well-known and widely respected community activist, Yolanda Garcia Park is a lively outdoor space for local families to gather, relax and play. The park includes modern playground equipment, an outdoor adult gym, a lush green room, and winding pathways for the Melrose community.
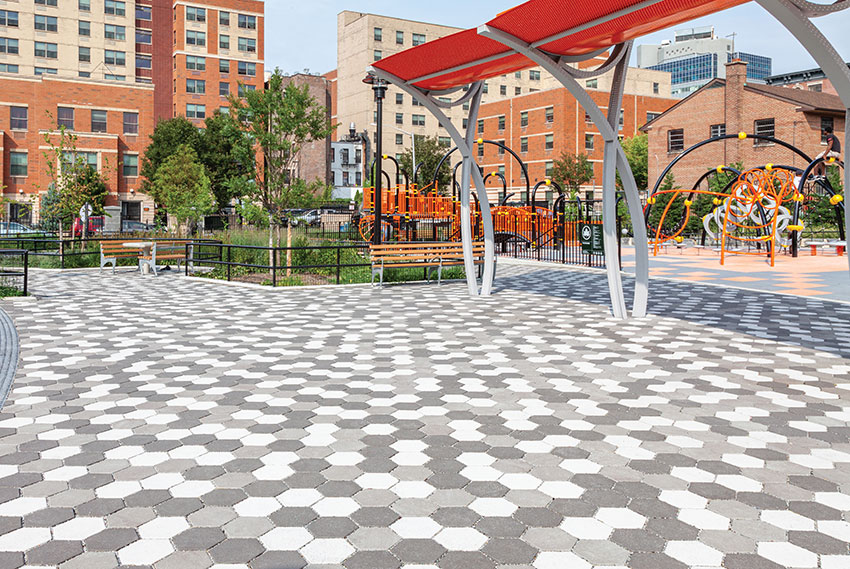
Photo courtesy of Unilock
Yolanda Garcia Park, Bronx, New York City.
New York City Parks incorporated permeable pavers into the design to meet the commitment to green infrastructure and sustainability. The unique hex paver shape in three distinct colors adds a timely urban feel to the space and creates a playful pixilated surface. The pavers’ ultra-smooth facemix finish offers the benefits of durability and long-lasting color, while the permeable application aids in reducing stormwater runoff. Capable of withstanding even vehicular loads, the paver provides maximum performance for active pedestrian walkways. What was once a vacant lot has been reimagined into a thriving center for active and healthy living.
Liberty Village, Toronto, Ontario
In Toronto, Ontario, the corner of this intersection creates a space to meet, rest and watch passersby. The permeable pavers used at the site do their part to help mitigate runoff in this well-aged community. The addition of the water feature and art installation provides a calming influence in a bustling city. PMA Landscape Architects designed it.
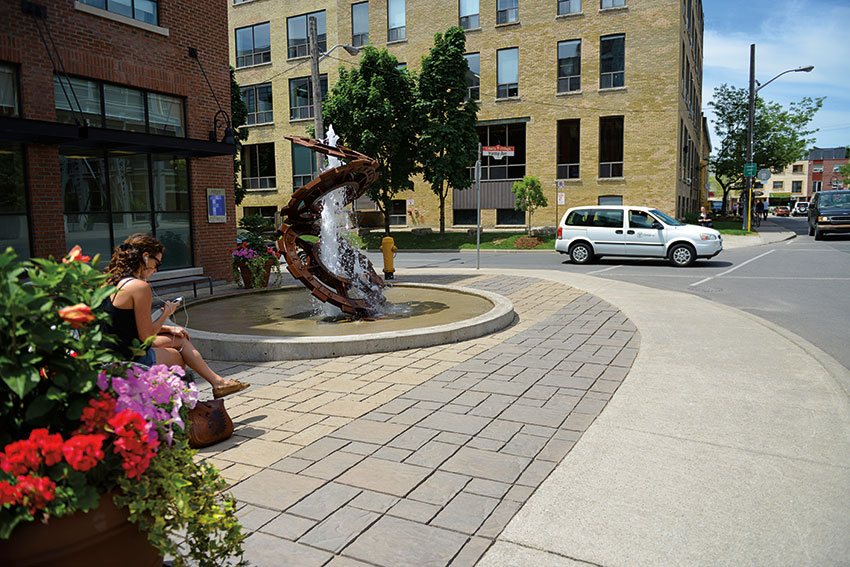
Photo courtesy of Unilock
Liberty Village, Toronto, Ontario.
Prairie District Park, Chicago
In Chicago, a pocket park replaces a vacant lot at the northeast corner of 21st Street and South Prairie Avenue. Designed by landscape architect site design group, ltd. (site) in collaboration with an active community group, the new park is divided into three parts: a playground with water sprayers for kids, a combination turf and pavement area for canines, and a plaza with seating. Permeable pavers were chosen for the park's walkways in a brown color to accent the nearby vintage brick lofts.
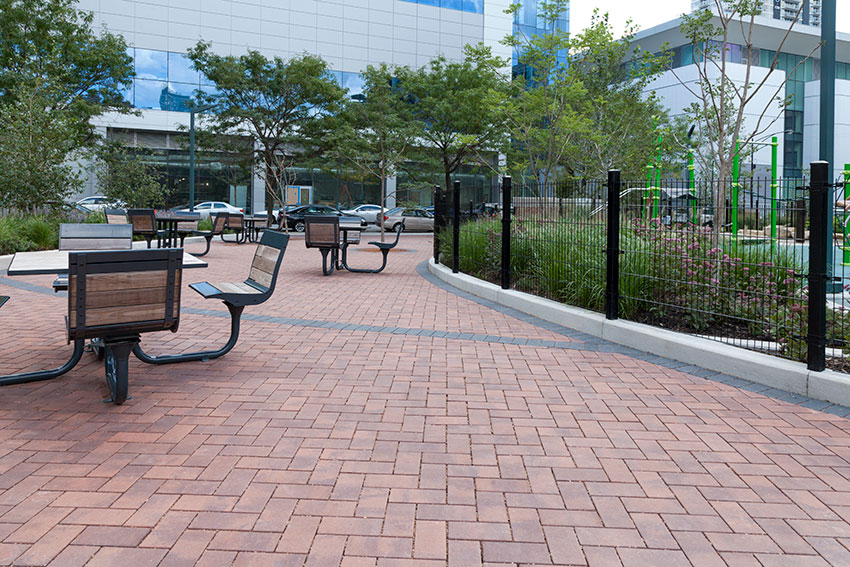
Photo courtesy of Unilock
Prairie District Park, Chicago.
The park is one of many components in redeveloping the McCormick Place area. It offers a welcoming and vibrant half acre of recreational space for the neighborhood to host community meet-ups and other programmable events.
Courtyard Park, Fulton Market, Chicago
Fulton West is a newly constructed and LEED Gold-certified mixed-use office and retail development located in one of Chicago’s hottest areas, Fulton Market. Serving as an architectural anchor of the neighborhood, the impressive nine-story building features a plethora of modern office amenities as it fronts onto a 20,000-square-foot courtyard park enclosed by the surrounding office buildings. The green space stands out amid modern aluminum and glass facades, creating unique visual interest and a welcoming environment for tenants. The lushly-landscaped courtyard provides an exciting gathering and event space, featuring a wide variety of colorful flowers and shrubs, custom wooden benches, water fountains, and a lawn amphitheater. Chicago-based site design group, ltd. (site) chose Unilock Permeable Plank pavers with Smooth Premier finish to create the walkways around the building entrance, providing a long-lasting, smooth, contemporary texture and alleviating any future stormwater management concerns that frequent the Chicago area.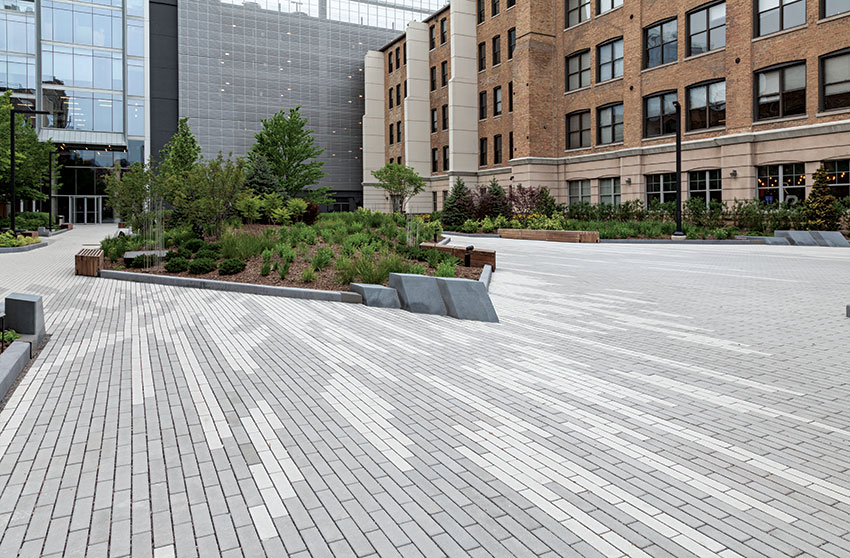
Photo courtesy of Unilock
Courtyard Park, Fulton Market, Chicago.
CONCLUSION
The design community continues pursuing net-zero carbon emissions for every project. Tangible goals are set for less than 10 years from now (2030), and much work must be done between now and then. Material developments, chemical advancements, and new methods are coming down the pipeline rapidly, retooling our resources for success. In the meantime, simple strategies can be used today—using products with recycled or carbon-friendly content, using materials that are already on site, selecting low-maintenance products, weighing the longer-term offsets of the product or system in which it is used, and designing carbon neutral projects that over time become net zero or and turn into carbon sinks. As these practices continue, we are heading to a carbon-neutral world.
END NOTES
1“Overview of Greenhouse Gases” U.S. Environmental Protection Agency. Last updated 13 April 2023. Web. Accessed 3 July 2023.
2“Carbon Footprint: Integrated Paving Solutions.” Portland Cement Association. Web. Accessed 3 July 2023.
3Jingjing Wang, Yurong Zhang, Yuanfeng Wang. “Environmental impacts of short building lifespans in China considering time value.” Journal of Cleaner Production. Volume 203. 2018. Web. Accessed 3 July 2023.
4Sanjuán, Miguel Ángel, Carmen Andrade, Pedro Mora, and Aniceto Zaragoza. 2020. "Carbon Dioxide Uptake by Cement-Based Materials: A Spanish Case Study." Applied Sciences. Web. Accessed 14 June 2023.
5Bezerra, J.V.A.; Mota, T.G.; Andrade, H.M.L.S.; Andrade, L.P.; Vigoderis, R.B. “Comparative study of retaining walls aiming to optimize carbon footprint.” Revista Ibero Americana de Ciências Ambientais. 2020. Web. Accessed 3 July 2023. .
6“Reduced Life Cycle Costs of Pervious Pavement: Permeable Pavement Provides Long-Term Cost Savings and Environmental Benefits.” North Central Texas Council of Governments. Web. 13 July 2023.
7“Permeable Interlocking Concrete Pavement Life-Cycle Cost Analysis Tools.” Applied Research Associates Inc. Prepared for Interlocking Concrete Pavement Institute. 31 Dec. 2018. Web. 13 July 2023.
8Bezerra, J.V.A.; Mota, T.G.; Andrade, H.M.L.S.; Andrade, L.P.; Vigoderis, R.B. “Comparative study of retaining walls aiming to optimize carbon footprint.” Revista Ibero Americana de Ciências Ambientais. 2020. Web. Accessed 3 July 2023.
Kathy Price-Robinson is a nationally known remodeling and construction writer. Her award-winning remodeling series, Pardon Our Dust, ran for 12 years in the Los Angeles Times. www.kathyprice.com