Building Resiliency
Designing buildings to withstand natural and man-made disasters through product specification
Continuing Education
Use the following learning objectives to focus your study while reading this month’s Continuing Education article.
Learning Objectives - After reading this article, you will be able to:
- List key natural and man-made disasters that affect the built environment.
- Define resilient design and how it benefits cities, communities, and the economy.
- Discuss how designing for resiliency can impact building design and improve occupant health, safety, and well-being.
- Describe the benefits of specifying products designed for resiliency.
- Explain how buildings can achieve a U.S. Resiliency Council (USRC) rating.
January 2018: Intense rainfall in Montecito, California, fell on land already scarred by wildfires. The loose soil on the bare land quickly turned to mud and produced massive mudslides that flattened homes and other buildings and covered local freeways. Gas lines ruptured and fires broke out. Twenty-one people were killed, and an estimated $208 million in commercial damages were sustained, with up to an estimated $204 million to rebuild or repair residential properties.1
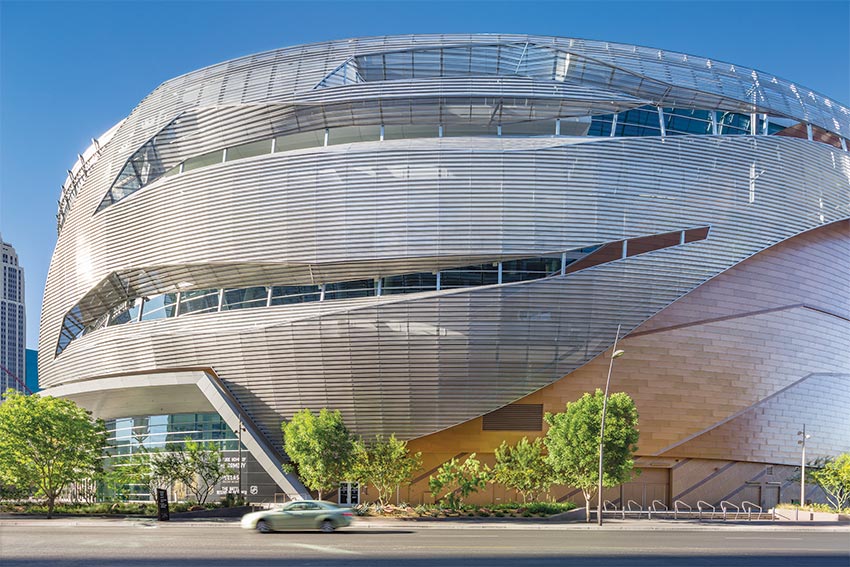
Photo: Lester Ali
Architectural louvers add a modern aesthetic to a building’s exterior while allowing air to move freely into its mechanical system. They can also be engineered to withstand severe weather events as well as accommodate fluctuating temperature extremes and pressure drops to ensure occupant comfort and safety.
May 2018: More than 8 inches of rain fell over the course of just a few hours in historic Ellicott City, Maryland, and created a river that rushed through the city. The flood devastated commercial and residential properties, swept away cars and people, and left the area in ruin, just two years after the city had suffered millions of dollars in damage and lost lives in an earlier flood.
September 2018: The large, slow-moving Hurricane Florence hit the Carolinas with high winds and record rainfall that fed into local rivers, which rose to record heights and flooded the areas. North Carolina suffered 36 inches of rain, with the Cape Fear River in Wilmington reaching a high-water mark of 8.27 feet.2 South Carolina got 24 inches of rain, with many regions suffering from floodwaters. Fifty-three people died as a result of the storm. Estimated damage totaled $24.2 billion.3
October 2018: Hurricane Michael, a Category 4 storm, made landfall in Florida with 150-mph winds and worked its way up to Georgia, which last had a major hurricane track inland in 1898. Michael continued up the Carolinas, which had been hit by Hurricane Florence the previous month, and then into Virginia. Recorded as the strongest storm since Hurricane Camille in 1969, Michael brought the strongest winds recorded in the region since 1992, when Hurricane Andrew flattened communities in Florida. Locations such as Panama City and Mexico Beach, Florida, were practically demolished. In Florida alone, 43 people were killed, and 10 more were lost in other affected states. The estimated damage came to $25.2 billion.4
November 2018: California wildfires flattened towns in both Northern California and Southern California. The Camp Fire blaze in Northern California destroyed more than 150,000 acres, including 130,000 homes and the entire town of Paradise. The fire is on record as the deadliest wildfire in California history. Between the fires, 106 people died, with at least 85 from the Camp Fire. The economic damage was upward of a record total of $24.2 billion.
This list of natural disasters is by no means exhaustive for the United States in 2018. Tornados, blizzards, hail storms, and extreme heat and cold events all impacted the country, racking up an estimated $150 billion in damages for the year. Data from almost the past 40 years shows a clear trend: severe storms are more common than they were even 10 years ago, let alone 20, 30, or 40. Droughts and wildfires have become increasingly common, and over the past 10 years, flooding has increased as part of the severe storm impact. Some of these weather events have become so powerful that they have literally destroyed communities; those communities will eventually need to rebuild and ideally will do so using intentionally resilient building designs that can help mitigate future hazards and reduce risks of human and economic loss.
Rebuilding devastated communities, however, is only one part of the challenge. Communities throughout the country are at risk of the impacts of natural disasters, and the disasters are not limited to weather-related risks. Parts of the country, such as California, are in seismic zones and thus can be damaged by earthquakes. And as news from around the world has shown, the U.S. infrastructure is by no means alone in being affected by storms and earthquakes; this is a global concern, and resilient building design can play an important role in protecting communities.
While so many of the buildings damaged or destroyed by storms, fires, and floods have been residential properties, we will be focusing on resilient design for commercial properties and retrofit projects. The point is not to minimize the importance of residential properties but instead highlight the opportunities in the commercial sector. These projects include, to a lesser extent, buildings that may be damaged by man-made disasters as well, such as industrial explosions.
Most Common Types of Natural Disasters and Hazards
Before we address the issues of resilient design, let’s look at the most common types of natural disasters and how they impact the built environment. Some of the following types go hand in hand, such as hurricanes and flooding; however, it helps to understand each in its own right.
Wind and Storm Events
Severe weather can come in many different forms, but the most common are:
- hurricanes;
- blizzards;
- ice storms;
- tornados; and
- damaging winds.
The above storm events all typically involve extremely high winds. In the case of hurricanes, blizzards, and ice storms, there is usually other damage such as flooding, which will be covered in the section on water inundation. For now, let’s look at the ways that high winds can impact buildings.
From a physical impact sense, high winds impose very high lateral (horizontal) and uplift (vertical) forces on buildings. Lateral forces, or high winds that impact the building, create differential pressure on the structure. Differential pressure refers to the difference in pressure between two points of a system. In the case of a building, that could mean two opposite sides, typically referred to as the windward and leeward sides. When differential pressure gets to be too high, as it can during hurricanes, it can deform or dislodge building materials, such as walls, windows, doors, and even structural beams. If you consider an extremely high wind blowing against a wall or roof and flowing over and around a building, you can imagine high pressure pushing against the windward side and suction pressure pulling along the leeward side. When these two forces combine, an uplift force may occur and pull tiles and sheathings off the roof. In extreme cases, it may even pull off the entire roof of a structure.
Structural damage from high winds depends on many different factors, from the wind speed—both sustained and gusts—to the building height and whether the building is shielded by nearby structures or topography. The building structure itself plays an important role in potential damage, mainly whether the frame, connections, and envelope have been designed to withstand high winds, but also factors such as the structure’s shape and whether windows, doors, and even vents are strong—but also protected.
In addition to damages caused by wind forces, wind-borne debris can also impact a building, particularly vulnerable features such as windows, skylights, doors, and even siding. Debris can be anything from materials dislodged from other buildings to those knocked loose by the wind. When wind-borne debris impacts a structure, it does so at a very high speed and can easily puncture the building envelope. Once this happens, the structure is at risk of water intrusion from the rain or subsequent flooding.
Floods and Water Inundation
Floods can happen due to many different reasons, from heavy rainfall over a short time during a hurricane or even a severe thunderstorm, to snow melt, dry soil, or storm surges. Three of the more common types of floods are coastal (surge flood), fluvial (river flood), and pluvial (surface flood).
Coastal floods are most often caused by severe weather, such as hurricanes, that produce high winds. These winds push the water onto shore—causing the water to “surge” onto the surrounding low-lying land. Coastal flooding can be exceedingly costly, especially if many industries and ports are located in the region.
Fluvial, or river floods, typically occur when rivers overflow after excessive rainfall, but they can also happen when heavy snow or ice melts upstream. Flash flooding is often associated with fluvial floods and is known to cause extensive damage because it carries debris along with the flood.
Finally, pluvial, or surface floods, are the product of heavy rainfall in areas not near a body of water. Often, these floods happen in areas where urban drainage systems cannot handle the flow or suburban development has left the ground unable to absorb the water. In both cases, water flows along streets, damaging buildings along its path. Pluvial flooding tends to happen at the same time as coastal and fluvial flooding.
In addition to these three basic types of floods, certain coastal areas are at risk of flooding from tsunamis or seiches. A seiche is a standing wave in an enclosed or partially enclosed body of water, such as a lake, bay, or harbor—but seiches can also happen in reservoirs and even swimming pools. Seiches behave much in the same manner as a storm surge along coasts, but because they are a wave in an enclosed body of water, they can move back and forth for a long time, causing flooding whenever they encounter the shoreline.
Regardless of the source, water inundation can severely damage buildings in several ways. First, in the case where a building is surrounded by water, the structure will be subjected to different forces. For example, standing or slow-moving water can push against the different sides of the structure, exerting hydrostatic forces. If the soil beneath the structure is saturated, a building may lift and float, as often happens with residential homes. Alternatively, hydrodynamic forces from fast-flowing water around the structure can erode foundations, pull off walls, and even dislodge a building from its foundation. As with high winds, rapidly rushing water can carry debris with it that can damage buildings. Finally, a lingering impact of floods is damage from eventual mold growth.
Climate-Related Events
Wildfires, drought, and extreme heat and cold events are all climate related, and they have all become increasingly common and more severe.
Wildfires are the most destructive, with impacts that can range from burning or melting building components to completely destroying towns, as was the case with Paradise, California. In addition to the immediate loss of the built environment, fires can cause power surges from damaged power systems, smoke and soot damage, and soil erosion—the latter of which can put the area at risk for flooding and mudslides.
While wildfires can start in any number of ways, from a carelessly tossed cigarette to an errant ember from an unattended campsite, the key is that they spread quickly and can impact structures within the region, usually via embers and firebrands, such as burning brush or trees, that get carried on air currents and land on building exteriors, which then ignite. Sometimes the embers or firebrands can enter a structure, for example, through an open window, and ignite the building from the inside. Regardless of the mechanism, once a wildfire impacts the built environment, it tends to spread rapidly.
Other climate-related events include droughts as well as extreme heat and cold events, which can impact the built environment through power outages (both hot and cold extremes), loss of thermal comfort, and loss of water sources. Extreme heat events occur when the temperatures are 10 degrees or more than the average high temperature and remain high for several weeks. Such events can trigger droughts, which have a cascading effect in communities, especially those with businesses that rely on agriculture.
Extreme cold events, such as polar vortexes, occur when the temperatures are at or below freezing for an unusually long period of time; for example, for several weeks. Sometimes, but not always, these cold events coincide with severe winter storms, which can knock out power supplies and leave buildings vulnerable to cold-related damage, such as burst pipes.
Geologic/Seismic Disasters
Finally, geologic and seismic disasters, such as landslides, mudslides, and earthquakes, can take a huge toll on the built environment.
Landslides and mudslides can both damage buildings caught in their paths. While similar in the sense that both are typically initiated by heavy rainfall in hilly regions where the soil cannot absorb the water and erosion has already occurred, the two differ. Landslides refer to the movement of earth and rock and occur on steep slopes, which crumble when the soil is destabilized. Mudslides, on the other hand, occur when an inundation causes groundwater to rise and the soil to lose friction. In either case, buildings can either be damaged as the moving earth impacts the building or as the soil beneath the building moves and shifts.
Earthquakes are another concern. The primary damage of earthquakes comes from ground shaking, with many different factors contributing to the damage, such as the strength and magnitude of the quake, how close a structure is to the fault, the local geology, and even the soil type. Ground shaking impacts buildings by moving them both laterally and vertically, both of which can cause the structure to fail from excessive deflection and deformation. In addition, earthquakes can often cause the ground to behave in ways that damage buildings situated on top of the zone. Given that buildings rely on a level, stable foundation, when the ground under them rapidly expands, or when the ground ruptures or the soil liquefies with a swell of groundwater or even suddenly sinks (subsidence), buildings can suffer.
In some regions, earthquakes are followed by tsunamis and flooding, and in other cases, the buildings may also be impacted by fires.
Current building codes for seismic resilience are designed to protect lives by specifying that buildings stay intact long enough for occupants to safely escape. In the event of a major seismic event, however, buildings may be damaged to such an extent that they are no longer safe for future use.
Man-Made Disasters
Natural disasters are not the only forces threatening buildings. Certain structures, particularly industrial sites, are also at risk of being damaged by man-made disasters, such as explosions. Facilities that produce or use combustible materials are especially at risk, and an explosion inside an industrial site can damage the building itself, all while putting the building occupants at great risk.
Regardless of the type of disaster, most also have cascading effects, whether they include fires after an earthquake, or power outages and infrastructure damage after a hurricane, ice storm, or blizzard. Such secondary effects only prolong the time it takes for a community to recover and rebuild. In terms of commercial spaces, this means businesses may be closed for longer, incurring yet another level of financial damage that may take months to recoup, if they manage to reopen at all. In the next section, we will look more in depth at the human and economic costs of natural disasters and, to a lesser extent, man-made disasters.
Resilient Design and How It Benefits Cities and Communities
Natural and man-made disasters happen. And we’ve seen that, at least in the case of natural disasters such as storms, floods, and wildfires, they are happening more often, and the damage is increasingly severe. Communities affected by natural disasters may suffer on a personal level from loss of life and property as well as income if businesses were affected. Depending on the disaster, roadways in and out of towns may be closed, disrupting the supply of goods and services, which can impact businesses lucky enough to avoid building damage. And the longer a business is out of service, the more likely it is to lose customers.
According to global insurance statistics, 40 percent of small businesses fail to reopen after they are affected by natural disasters simply because they cannot afford to repair the buildings. Moreover, 25 percent of the businesses that do reopen tend to fail within the year as long as they weren’t impacted for longer than five days; the longer they were impacted, the less likely they are to succeed. The U.S. Small Business Administration notes that up to 90 percent of businesses that were closed for five days or more are likely to fail within the first year of opening, and 75 percent close their doors within two years.5
But small businesses aren’t the only buildings to be affected when disaster strikes. Other commercial structures, such as office spaces, sports complexes, and aviation and transportation complexes can all impact the daily life of the community if they are out of commission. Even more critical are public structures such as schools, libraries, first-responder stations, jails, hospitals, and senior-care facilities, all of which provide critical services, especially in times of need. When such structures are not able to meet the needs of the community, other buildings must be able to pick up the slack. Hurricane Katrina comes to mind, for example, where the Superdome in New Orleans was used as a makeshift shelter for people who had to evacuate their homes. When conditions there became unmanageable, people were bussed to the Houston Astrodome, which housed nearly 25,000 hurricane victims.
Again, Hurricane Katrina serves as a good example of the problems that can arise with the cascading impacts from a natural disaster. In this case, hospitals were critical during and after the storm, and for the most part, many only suffered superficial damage from the storm. However, the cascading impacts on the infrastructure meant that, like many other buildings in the city, they had to be evacuated because of loss of power, water, and sewage services. In the days following the storm, 11 area hospitals were inaccessible because of the floodwaters, with a total of 1,749 patients in care. Because of the nature of the disaster, the hospitals also took in people who left their homes; the Louisiana Hospital Association (LHA) estimates that the hospitals housed more than 7,600 people in addition to the patients during the immediate aftermath of the storm.6
Examples such as Katrina show the extremes, but in the almost decade and a half since the hurricane, we have seen so many different versions of the extremes, whether through storms, fires, floods, or other disasters. Each time, vulnerable structures fail, citizens are displaced, and buildings that can withstand the disaster shelter those in need.
This brings us to the heart of resilient design: buildings designed to withstand weather extremes, seismic forces, or the impacts of natural and man-made disasters tend to do their job and do it well. Resilient design is an opportunity for developers, architects, and engineers to engage in measures that can protect lives and property, extend the lifespan of buildings, reduce the number and amount of post-disaster repair costs, and lessen the down-time before buildings can return to their necessary function.
Resilient Design: What It Is and Why It Matters
To fully understand resilient design, it’s important to understand what we mean when we talk about “resilience” in the building profession. According to the Resilient Design Institute, resilience is “the capacity to adapt to changing conditions and to maintain or regain functionality and vitality in the face of stress or disturbance. It is the capacity to bounce back after disturbance or interruption.”7 If we go back to the list of natural disasters and various secondary impacts discussed in the previous section, we see that this definition covers not only the built environment but also the surrounding communities and infrastructure. How long does it take for a town hit by a tornado, or a hurricane and flood, or a wildfire, to recover and get back to regular daily life? A built environment that is not resilient can take a long time to recover; however, a place that is resilient may suffer less damage and therefore be able to bounce back more quickly.
With that in mind, resilient design refers to buildings, landscapes, communities—and even regions—that are deliberately designed to withstand impacts that might otherwise damage or destroy the community. As with many health, safety, and welfare decisions, resilient design stems from seeing how vulnerable buildings and communities have fared in different disaster situations and turning those lessons into practical solutions.
Often, communities greatly affected by natural disasters shift their priorities to resilient design. Buildings in seismically active areas, for example, may be constructed to sway when the ground shifts or be built from materials that resist the secondary issue of fires. The same goes for areas that are often in the path of hurricanes or other severe storms, with design focusing on mitigating water intrusion and wind damage.
Resilient design relies on three key factors: hazard mitigation, passive survivability, and adaptation. Combined, these factors can help buildings, and thus communities, become more resilient against the impacts of natural and man-made disasters. Buildings and communities designed with resilient practices and materials can also be protected against the financial costs of damage, and thus play a role in benefitting the local and broader economy as well.
The Federal Emergency Management Agency (FEMA) defines hazard mitigation as “any action taken to reduce or eliminate long-term risks to people and property from natural disasters.”8 By extension, hazard-mitigation planning is “a process used by state, tribal, and local governments to identify risks and vulnerabilities associated with natural disasters and develop mitigation strategies to reduce or eliminate long-term risks.”9 Mitigation planning measures tend to stem from previous disasters and the lessons learned—and in terms of the built environment, those lessons are incorporated into newer versions of building codes. New lessons are being learned every time a new disaster happens, and mitigation strategies continue to improve. As a result, new structures often take into account factors such as roofs designed in shapes that have high-wind tolerance, rainwater management from roof run-off, or even slab-on-grade construction that eliminates basements (prone to flooding) in the first place.
As a way of keeping first responders and building professionals up to date, FEMA provides extensive programming and resources that address issues concerning communities as well as individual building strategies, available at www.fema.gov/hazard-mitigation-planning.
Passive survivability refers to a building’s ability to maintain the basic functions critical to keeping occupants safe and comfortable, specifically when power, heating, and/or water are lost for an extended time. Often, schools, hospitals, and civic buildings are designed with more passive survivability features than the average residential house, and as such they are often used as emergency shelters during disasters.
Adaptation in terms of building design considers mitigation over time, and the assumed changes and evolution of both building design and community responses. Buildings can be designed to include adaptive strategies that anticipate impacts of future natural disasters.
Resilient building design benefits cities, communities, and the economy by protecting lives and property, extending the lifespan of buildings, reducing the number and cost of post-disaster repairs, and minimizing building downtime.
Designing for Resiliency: Architectural Products
Specialty architectural products are at the core of resilient building design. Most of these products tend not to be high-profile from an aesthetic sense and may initially be seen as minor design features, but the role they play in keeping buildings safe and secure for occupants can be significant. That said, architectural louvers, which we will discuss, are coming into their own in building design with some elegant and functional styles that allow for architectural creativity. This section will discuss key architectural products designed to address a wide range of natural and man-made disasters, protect buildings, and keep occupants safe.
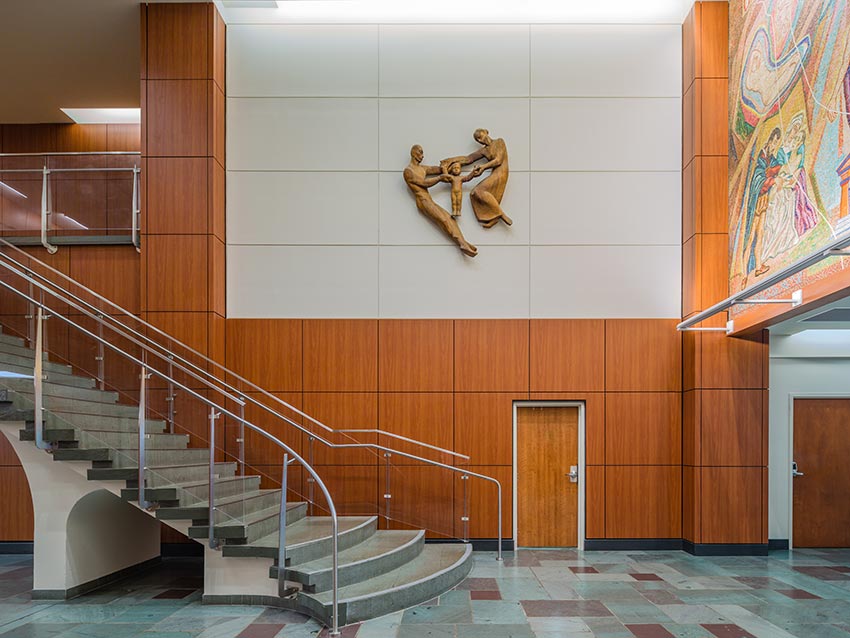
Photo: Ted Miles Photography
Resilient buildings include strategic interior design, as shown here at the University of South Alabama Medical Center. Hospitals and other public facilities can be gathering spaces after natural or man-made disasters, and they need to withstand heavy pedestrian traffic and abuse from carts, gurneys, and other bumps and scrapes. Pre-engineered wall-protection systems offer unmatched durability and impact resistance to preserve the built environment and protect capital investments.
Expansion Joint Covers
Expansion joints, also known as movement joints or MJs on structural drawings, are structural gaps designed to accommodate the movement of a structure or building in a controlled manner, thus protecting the internal and external finishes from potential damage. Movement may come from one or several different sources, including:
- thermal expansion or contraction of the building material as it responds to changes in temperature and moisture (swells and contracts).
- building settlement from live and dead loads of the structure on its supporting foundation.
- wind sway, as caused by the effect of strong winds on a building. The taller the building, the more significant the sway.
- seismic activity, which is a multidirectional movement, such as that caused by earthquakes.
Structures in dense urban areas may also experience vibration from local traffic or subway trains.
Many different structures can have expansion joints, such as bridges, sidewalks, and even ships—any structure where movement needs to be controlled. In buildings, the joints run through the building—both laterally and vertically along floors, ceilings, and walls—and are often wider near the top of the structure.
Expansion joint covers (EJCs) are designed to cover the gap of the expansion joint with minimal interruption to a building’s aesthetics and are engineered to accommodate any of the four types of movement previously mentioned. In seismic zones, expansion joints are implemented to allow the building to move with little to no damage during a seismic event. Moat cover systems accommodate very large movements around the perimeters of base-isolated structures. These systems are critical for the safe and functional egress of a building’s occupants and ingress for first responders during and after an event.
EJCs provide a covered transition across the expansion-joint opening and are not affected by movement along the surfaces of either side of the joint. They are a standard component for all large plan buildings; however, every building design is different in how the EJCs are used according to different performance requirements. Floors in different structures, for example, may require different types of joints and joint covers. In high-traffic buildings, such as shopping malls or airports, the joints and covers will need to resist the wear and tear of constant use, and so strong metal covers are appropriate. In a hospital, however, where infection control and patient comfort are critical, a flush floor finish and rubber seal is required to minimize jarring as gurneys and wheelchairs are rolled down hallways.
From a practical standpoint, it is important to specify the correct EJC model based on nominal, maximum, and minimum joint-size opening, which is typically determined by an engineer. Loading is also a critical component to ensuring the system functions correctly on a day-to-day basis. If these two factors (size and loading) are not correct in the specification, the EJC may not be installed correctly and will not function properly as needed.
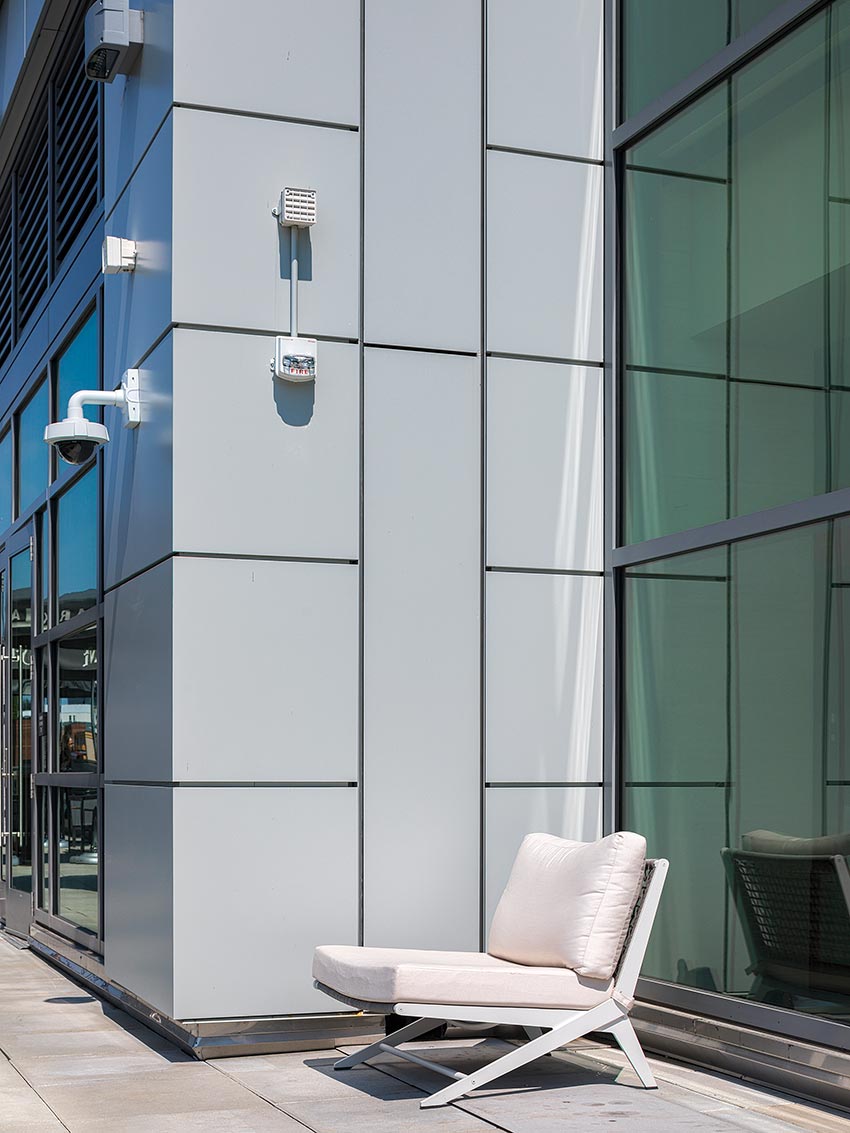
Photo: Sozinho Imagery
Expansion joints help stabilize buildings during freeze-thaw events and earthquakes. Lincoln Square, a mixed-use development in Bellevue, Washington, called for expansion-joint covers throughout the commercial and residential development to protect against shifting ground and building sway that can occur during seismic events.
Photo: Sozinho Imagery
Expansion-joint covers range from standard solutions for floors, walls, ceilings, and roofs to highly engineered systems for corridors, base isolations, and stairs. They protect structures against damage and cracks that can occur during ground settling, thermal expansion and contraction, wind sway, and seismic activity. Some provide fire- and vapor-barrier protection.
EJCs help make buildings more resilient by addressing the different potential sources of movement listed above. For example, in buildings where freeze-thaw is an issue, or in areas where temperature extremes are common, the joint will help ensure that the building structure does not crack. Likewise, in regions where strong winds or hurricanes regularly occur, the joint and joint covers will protect the building as it sways. The same holds true for seismic activities.
Architectural Louvers
Architectural louvers are shutters or window blinds with adjustable horizontal slats that promote daylighting and airflow into a building but keep out precipitation and sunshine. As a design feature, architectural louvers can be used to cover building facades and equipment. Louvers are typically found where a building’s mechanical rooms are located, usually on top of the building or on mechanical floors around the building. They allow proper airflow so that the mechanical equipment can function properly, yet they still protect the equipment from wind-driven rain that occurs, especially during hurricanes.
From a resiliency perspective, architectural louvers can be used to resist high winds and rain, with some designs built to withstand hurricane and tornado conditions. In such extreme weather conditions, it is important that a building maintain airflow without water intrusion, which can happen if a louver isn’t designed to resist and rapidly drain water. In situations where a potential disaster may be man-made, such as an explosion, architectural louvers designed to be blast resistant can help protect the building and its occupants. Specifically, these louvers are designed to remain in their frame and stay anchored in the event of a blast so that the louver itself does not become a projectile.
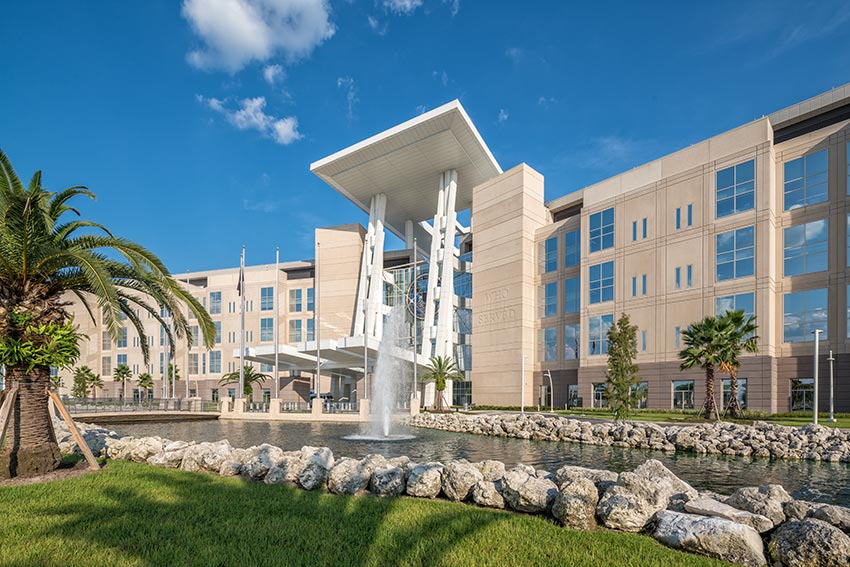
Photo: Ken West Photography
The Orlando Veterans Affairs Medical Center includes blast louvers engineered to withstand explosions up to 12 psi. The blast louvers are AMCA certified and designed to remain in place during explosive events so they don’t become a projectile—a government requirement.
Photo: Lester Ali
The Penn Medicine Smilow Research Center includes storm-resistant louvers that protect its mechanical systems against wind-driven rain and severe winter storms, including bomb cyclones. Louvers are engineered and tested to perform as intended during various weather conditions, including rapid temperature fluctuations and pressure drops.
Explosion Relief Vents
Explosion relief vents are safety devices built into industrial buildings where excessive internal, explosion-incurred pressure is a risk; the vents work as a pressure relief. Explosion relief vents can help a building release pressure and survive a natural or man-made disaster involving explosions and excess internal pressure. Industries where combustible dust, gas, or chemicals are manufactured or used in manufacturing other products are at particular risk for explosions, which can be deadly to industry workers and impact the surrounding communities.
A key requirement of explosion relief vents is that they must be extremely strong to ensure that they remain intact and do not present a risk of pieces breaking off and becoming projectiles. Consequently, it is very important for explosion relief vents to be designed to meet National Fire Protection Association (NFPA) guidelines and to meet all relevant International Fire Code (IFC) building codes. Some vent designs can be tested and are easily reset after an explosion event.
Explosion relief vents are critical not only for protecting a structure during an explosion but also for keeping the building occupants and local communities safe from the potential impacts of an explosion.
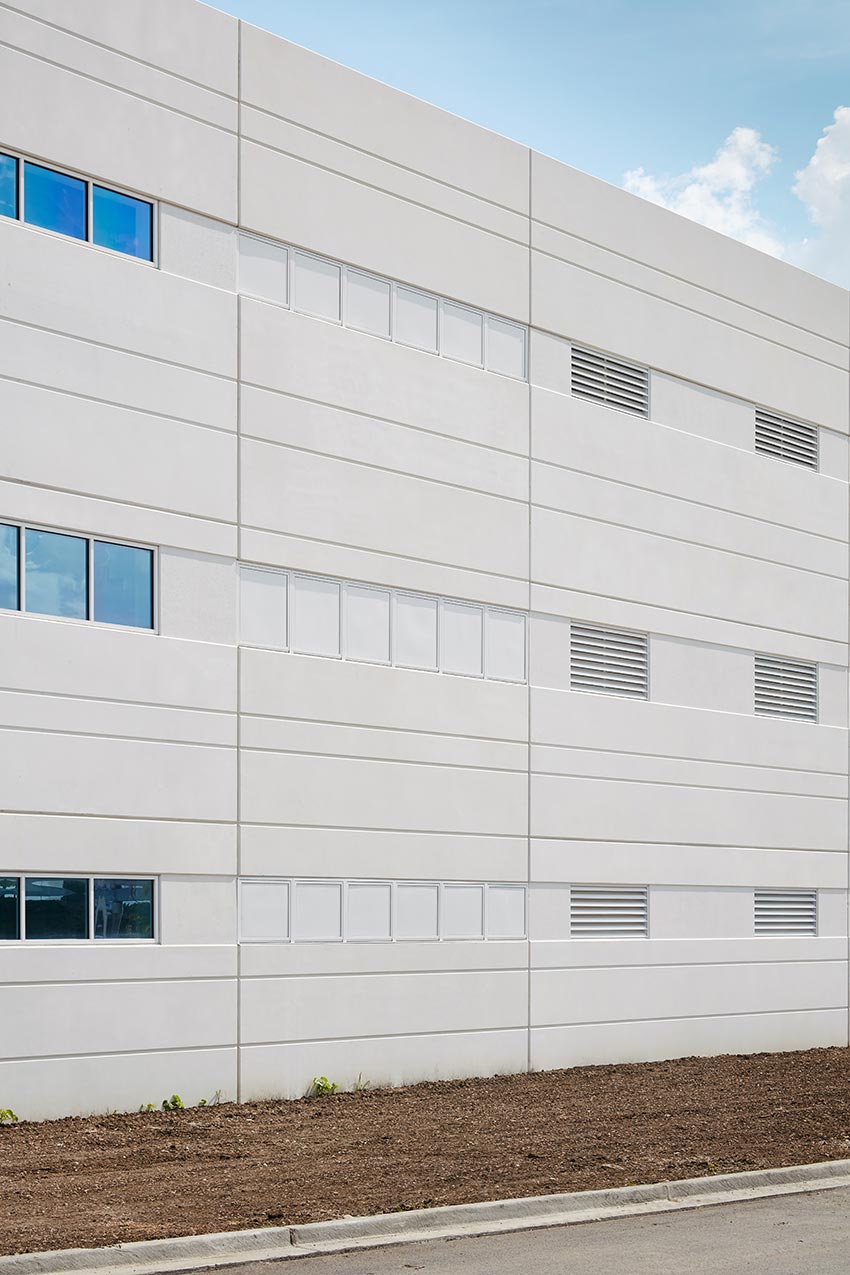
Photo: Dustin Halleck Photography
The Chicago O’Hare Consolidated Rental Care Facility features explosion-relief vents designed to protect life and property in the event of smoke, fire, or an explosive blast.
Interior Wall Protection
In many cases, when we talk about resiliency, we think about how the external elements of a building are protected from weather extremes and other natural disasters. But resiliency also applies to the everyday use of interior spaces, such as high-traffic buildings like schools, hospitals, hotels, airports, and offices. In such spaces, the interior walls can be easily damaged by everything from machinery and heavy equipment to building occupants and pedestrians. Interior wall protection features, such as rigid sheet panels, corner guards, wall-protecting crash rails, door and frame protection, or even ergonomic handrails, can improve a building’s resiliency on a day-to-day basis and help minimize repair and maintenance costs while adding a customized aesthetic pop to a space. Certain features, such as rigid sheet coated doors, enhance fire resistance and are designed to meet the strictest fire codes, thus enhancing occupant safety in the event of a fire.
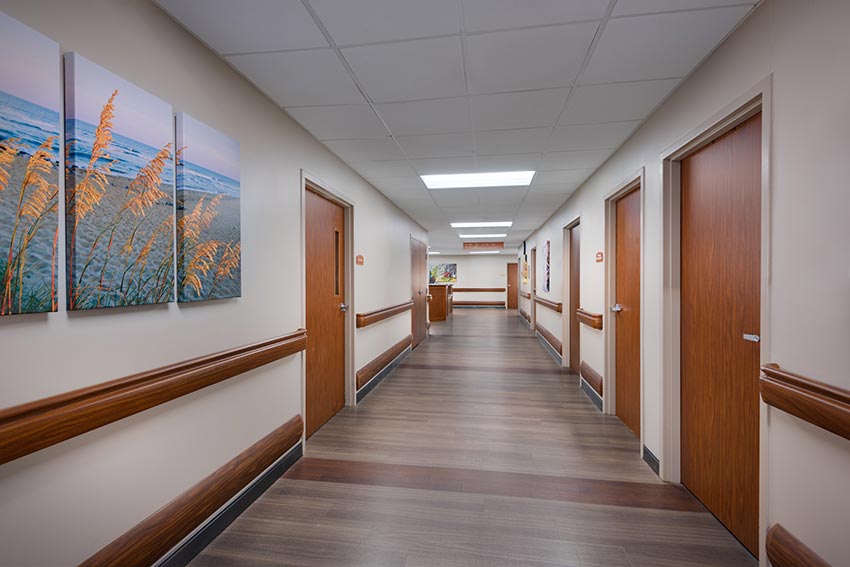
Photo: CPI Productions
Health-care facilities that utilize wall-protection products can withstand heavy use and abuse without showing wear and tear.
Photo: G. Lyons Photography
Interior wall-protection products are durable, impact resistant, and easy to clean to keep facilities looking good with minimal maintenance.
Photo: Joe Aker
Sugar Land Rehab Hospital in Sugar Land, Texas, incorporated interior wall protection into its design to enhance the beauty and durability of patient rooms. The wall protection is durable enough to hold up to the frequent cleaning requirements of the hospital’s environmental services staff.
Entrance Mats and Grids
Entrance mats and grids, while an often-overlooked feature of a building, are key in keeping building occupants safe while reducing the required maintenance of a building. They are designed to trap dirt, sand, and water so that the floor beyond the entrance remains safe, clean, and presentable. Entrance mat systems address the specific needs of high-traffic areas where building occupants are likely to bring in dirt and debris from the outdoors. Such mats are designed to scrape off dirt and trap it within the mat for future cleaning. Entrance grids can also be installed in high-traffic areas in or near building entrances to perform a similar dirt-catching function as mats, but they tend to trap and store more dirt than a mat. A stainless-steel grid is another option that requires almost no maintenance once it is installed and can be highly effective in protecting flooring from tracked-in dirt and moisture—thus protecting building occupants from potential slipping. The frequency of cleanings will depend on the amount of traffic a building sees on a daily basis and weather conditions that occur. Entrance mats and grids must be durable for cases when heavy machinery like scissor lifts, dollies, or vending machines need to enter or exit the building.
Entrance mats and grids aid in building resiliency by keeping the buildings clean and safe for occupants and also helping keep hallway floor surfaces free of water, thus preventing slip hazards.
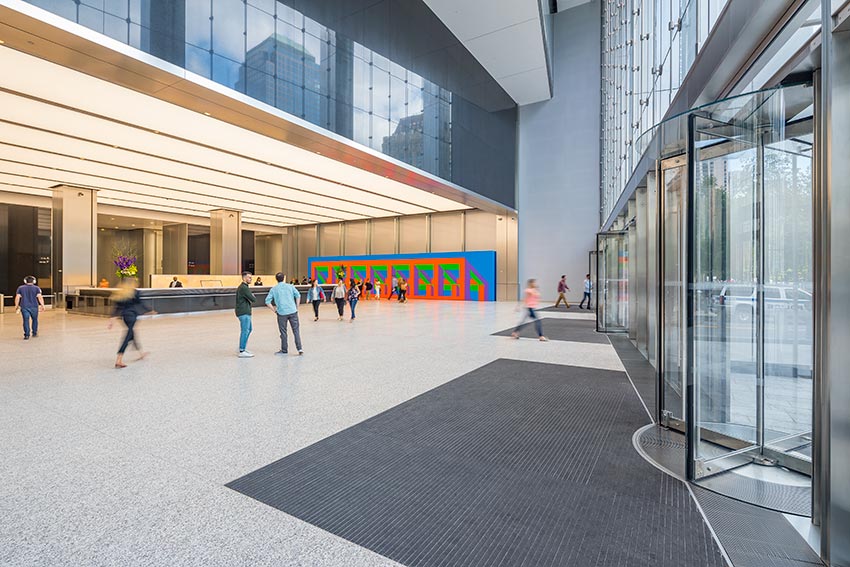
Photo: Lester Ali
Entrance flooring solutions stop debris at a building’s entrance, as shown here at 3 World Trade Center in New York City. The right solution will trap dirt, snow, slush, and ice to reduce maintenance costs and protect the safety and security of occupants.
Designing for Resiliency
When we take a minute to step back and look at the risks associated with not designing for resiliency—such as the loss of life, extensive damage to property, and long-term economic toll on communities and businesses—it makes sense to incorporate some resilient design strategies whenever possible. Everything from being aware of the potential risks for the local environment to the specific topographical location can help. For example, is a project in a region known to flood? Or even if a flood hasn’t happened recently, are there rivers nearby that may present a risk? Are fires a hazard? Earthquakes? By taking the time to plan, design, model, and build for the possibility of extreme weather events and their impacts, architects and engineers can work together to create beautiful, resilient buildings that have a better chance of withstanding 100-year storms that occur year after year. In doing so, these buildings can help communities and businesses get back to work more quickly and with less financial strain. The first step in this process, however, is choosing architectural products designed for resiliency and incorporating them into the design early in the process.
Testing, Standards, and Ratings: The U.S. Resiliency Council (USRC)
Rating Systems
The U.S. Resiliency Council (USRC) has established a building rating system that assigns buildings one to five stars based on the dimensions of safety, monetary damage, and recovery time to regain basic functionality after an event.
Within the rating, safety focuses on the potential for occupants to safely escape the building unharmed. The damage rating concerns the estimated building repair costs after an event as a percentage of the building’s replacement costs. The recovery rating is expressed as how long it will take a building to regain basic function. This is an estimate of the minimum time required to repair and remove safety hazards from the building. In regard to earthquake hazards, the ratings are based on how intense the ground shaking is in terms of local building code requirements for a new building. At this point, there are no ratings for other hazards such as wind, wildfire, and flood; however, the USRC is in the process of developing them.
From highest to lowest, the rating categories are Platinum, Gold, Silver, and Certified, and they are detailed below according to USRC.10
The USRC Platinum rating represents the highest level of building performance and is intended to exceed modern code standards in terms of safety by protecting occupants against major injury and egress restrictions. Platinum-rated buildings are expected to suffer negligible damage (less than 5 percent of replacement cost) and allow functional recovery within a few days of a major seismic event. USRC Platinum is sought by owners who demand the highest level of asset protection and virtually uninterrupted functionality of their operations.
The USRC Gold rating represents a very high level of performance that is intended to exceed modern code standards in terms of safety by protecting occupants against major injury. Gold-rated buildings are expected to suffer only minor damage (less than 10 percent of replacement cost) and allow functional recovery within several weeks of a major seismic event. USRC Gold is sought by owners who demand high levels of asset protection and minimal disruption to their operations.
The USRC Silver rating is for buildings that, in addition to meeting the Certified standards, are expected to suffer significantly reduced damage (less than 20 percent of replacement cost) and allow functional recovery within a few months of a major seismic event. USRC Silver is awarded to buildings where limiting damage is an important consideration, such as for properties with commercial loans.
The USRC Certified rating is for buildings that have been evaluated by the USRC and comply with modern codes for performance in earthquakes. Certified buildings are expected to perform in a manner that will preserve the life safety of the occupants, limit damage to repairable levels under 40 percent of replacement cost, and allow functional recovery within a year of a major seismic event. Nearly 60 percent of most cities’ existing building inventories will not comply with this standard. USRC Certified signifies that a building is expected to achieve a level of performance consistent with new building standards.
Manufacturers and companies that rely on USRC ratings to certify their products understand just how important their contributions to resiliency efforts are, and the role they play in making communities safer and better able to withstand the impacts of natural and manmade disasters. As Gabriel Blasi, senior general manager at Construction Specialties, notes, “Our commitment to preparing our built environment to cope with natural disasters will be the platform on which we protect humanity and the places they frequent. The USRC can make this reality, and we are proud to support their efforts.”
Tests and Certifications for Resilient Building Products
Given the importance of resilient building design, the architectural products used in projects must meet the strictest performance standards. Testing is the first step toward ensuring that a performance standard has been met. Each architectural feature used in resilient design must pass key tests to ensure that it can perform above and beyond in extreme conditions. Let’s look at some of those tests.
EJCs are tested to ensure they will perform in a seismic event. Specific tests include the Seismic Corridor System (MACC) Cycle Test, Seismic Exterior Wall System (XLS) Cycle Test, Seismic Moat Cover Systems & Corners Cycle Test, and Seismic Tread and Riser Cycle Test.
Architectural louvers are tested and certified by the Air Movement and Control Association (AMCA), which is a third-party testing agency for the louver industry that tests and certifies louvers for air, water, and impact performance. AMCA 540 is a test method for louvers impacted by wind-borne debris for which the louver cannot become dislodged from the opening. This test ensures that the louver itself does not become a projectile. AMCA 550 is a test method for high-velocity wind-driven rain-resistant louvers and is tested to the following conditions: rainfall rate of 8.8 inches per hour tested to 35, 70, and 90 mph wind-driven rain for 15 minutes, and 110 mph wind-driven rain for 5 minutes. During all four tests, only 1 percent of total water sprayed is allowed through. According to AMCA 550, louvers that protect air intake openings in structures located in hurricane-prone regions, as defined in the International Building Code, shall comply with AMCA 550.11
Interior wall-protection products and materials must meet governing building codes, such as the International Building Code (IBC) and National Fire Protection Association (NFPA), which mandate that all building materials meet the minimum of a Class C/3 rating.
Products such as handrails, crash rails, bumper guards, accent rails, corner guards, and wall coverings must be UL classified and labeled Class A/1. Fire-rated, flush-mounted corner guards should be UL labeled with 1-and 2-hour installation. Wall systems should be UL classified and labeled Class B/2. Doors should aim for being Intertek labeled for 20-minute wood fire doors and 60-minute wood mineral core fire doors with noncombustible stiles and rails. Class A/1 designates the material’s surface-burning characteristics when tested in accordance with UL723, ASTM E84, and CAN/ULCS101.2.
For explosion relief vents, fire and smoke vents must meet UL standard 793 for smoke and heat and should be IBC and IFC Section 910 and NFPA 204 compliant.
Entrance mats and grids must stand up to rolling loads.
Conclusion
It’s safe to say that extreme weather events and related natural disasters are concerns that need to be taken very seriously. Throughout the United States and the world, cities and communities have been devastated by the impacts of hurricanes, floods, fires, wind, and ice storms, along with other natural disasters such as tsunamis and earthquakes. As many communities struggle to rebuild and others wonder if they’re next, architects, engineers, and developers all have an important opportunity to ensure that the structures they design and build fully embrace resilient building techniques and use architectural products that can help them achieve resilient outcomes.
End Notes
1Robert D. Neihaus Inc. “Preliminary Impact Assessment: Montecito Mudslides.” March 2018. Web. 18 April 2019.
2Shapiro, Emily. “5 Natural Disasters That Devastated the US in 2018.” ABC News. 8 Dec. 2018. Web. 18 April 2019.
3“Billion-Dollar Weather and Climate Disasters: Table of Events.” National Oceanic and Atmospheric Administration. National Centers for Environmental Information. Web. 18 April 2019.
4Ibid.
5Brown, Stephen. “Business Continuity Planning: The Often Under Prioritized Responsibility.” International Facility Management Association. March 2016. Web. 18 April 2019.
6Gray, Bradford H. and Hebert, Kathy. “After Katrina: Hospitals in Hurricane Katrina. Challenges Facing Custodial Institutions in a Disaster.” The Urban Institute. July 2006. Web. 18 April 2019.
7“What Is Resilience?” Resilient Design Institute. Web. 18 April 2019.
8“What is Hazard Mitigation?” Federal Emergency Management Agency. Department of Homeland Security. Web. 18 April 2019.
9Ibid.
10“Understanding Your Building’s Performance in Disasters.” U.S. Resiliency Council. Web. 18 April 2019.
11Kelechava, Brad. “AMCA Lover Standards.” American National Standards Institute. 18 May 2017. Web. 18 April 2019.
12Penndorf, Jon. “Adapting Building Design to the Effects of Climate Change.” Urban Land Magazine. 10 Aug. 2018. Web. 18 April 2019.
13“RELi 2.0: Rating Guidelines for Resilient Design + Construction.” U.S. Green Building Council. December 2018. Web. 18 April 2019.
14“About Us: Our History.” Zuckerberg San Francisco General Hospital. Web. 18 April 2019.
Rebecca A. Pinkus, MTPW, MA, is a Toronto-based communication consultant, writer, editor, and historian of technology. She has been writing for the green build industry for several years, contributing to more than 40 continuing education courses and publications through Confluence Communications.