Who’s the Culprit in WRB-AB Leakage?
Overcoming issues with integrated sheathing
Continuing Education
Use the following learning objectives to focus your study while reading this month’s Continuing Education article.
Learning Objectives - After reading this article, you will be able to:
- Explore how fasteners attached to sheathing become a point of vulnerability during extreme weather events, which can stress wall assemblies and cause water to enter the building envelope.
- Review the differences between standard test methods and the extreme conditions that were introduced by RDH Building Science in order to test several fastener options.
- Understand the differences between thin- and thick-mil fluid-applied barriers versus integrated WRB-AB sheathing solutions. Know which option performed best when exposed to high-wind and heavy-rain simulations.
- Discover best practices for cladding attachment options, depending on factors such as climate, building form, and architectural complexity, among others.
Extreme weather events can stress wall assemblies, causing water to enter the building envelope. Fasteners attached through sheathing are a point of vulnerability requiring specific attention in order to avoid leaks and compromising building integrity. With some minor exceptions, the standardized test methods for water-resistive barriers (WRBs) and air barriers (ABs) do not consider the range of adhesive and mechanical cladding attachments. These standardized methods also do not include simulations of extreme wind and rain on the cladding attachment penetrations. In this course, we will examine parameters and outcomes of water penetration testing, specifically on cladding attachments using ASTM E331 methodology. The intent of this testing is to determine the ability to resist liquid water penetration of multiple attachment clips in three different WRB-AB configurations under varying conditions. The WRB-AB configurations include thin- and thick-mil fluid-applied barriers over glass mat gypsum sheathing and an integrated sheathing system, which integrates the WRB-AB into the fiberglass mat and core. Testing simulated extreme water and wind that pushed the tested WRB-ABs to the point of failure. The results demonstrate how the tested WRB-ABs performed with the main source of leakage occurring at the fasteners. Based on this information, best practices for addressing cladding attachment penetrations are offered under different scenarios and with specific climate and construction risk factors considered.
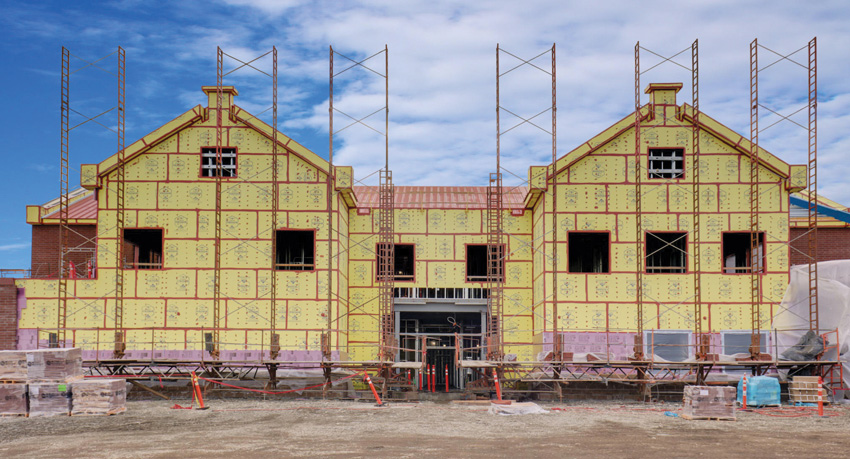
All images courtesy of Georgia-Pacific Gypsum
Exterior sheathing over conventional framed wall construction in commercial buildings needs to include a proven water-resistive barrier (WRB) and an air barrier (AB).
The Issues
Determining the relevant issues is the logical starting point when investigating any construction assembly, such as exterior walls in commercial buildings, which are the focus here. Specifically, we will look at known issues such as how to assure that WRBs and ABs remain continuous across changes in the construction. We will also address the importance of drying capability in a wall since it is reasonable to expect that water may penetrate in some form at some point in the life of the building. The remaining issue is focused on the variability of some common construction techniques that are used in exterior wall construction, including the impact of attaching cladding over a continuous WRB-AB. All of these are discussed further in the following sections.
Continuous Barriers
Framed exterior walls, using wood or metal studs, headers, etc., commonly use exterior sheathing secured to that framing to serve as the base or substrate for additional materials to be applied over it. Because of the need to be durable, consistent, resistant to fire, and easy to install, fiberglass mat-faced gypsum board has become one of the most popular choices for this type of sheathing on commercial buildings. Building and energy codes require that both a WRB and AB be present, and common practice today frequently sees these systems installed on the exterior face of the sheathing once it is in place. The WRB is to protect the rest of the construction and may also serve as a drainage plane to allow moisture or bulk water to drain away. The air barrier is to prevent uncontrolled air movement. Uncontrolled air movement can result in unnecessary energy consumption and also carry water vapor and/or pollutants through the wall assembly, which may have deleterious effects on the wall assembly and the building occupants.
Architects have choices in the ways that both the WRB and AB can be provided. We will look at four of the most common ones for commercial construction: liquid membranes that are rolled or sprayed onto the sheathing, self-adhered sheet membranes, foam plastic insulation, and integrated sheathing, which incorporates the WRB and AB directly into a sheathing product.
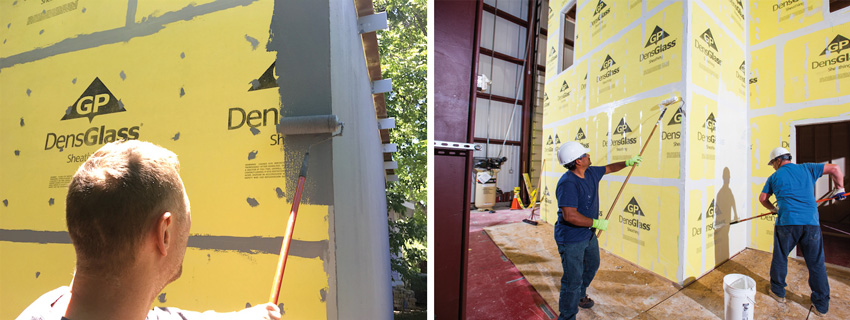
Liquid-applied barriers can be rolled or sprayed over exterior sheathing to create a continuous WRB-AB.
Liquid membranes: Continuous membranes that are liquid based are often used to provide either a WRB, an AB, or, in some cases, one liquid will provide both. They are installed at the job site either by spraying or rolling by hand, and to be effective, they must cover the gypsum board sheathing completely. The liquid nature of these membranes often makes them appealing because they can readily cover any irregular shapes or surfaces in the wall construction. However, since they are hand-applied, the quality of the installation is directly subject to the skills of the applicator. They also need the proper equipment, whether they are rolled on or sprayed on so that their application is uniform and consistent. It is important to note that each such product is tested for its effectiveness based on its final membrane thickness. Hence, some products need a measurably thicker and consistent application than others to achieve a full WRB-AB performance level.
At first glance, it is easy to assume that roll-on and spray-on liquid barriers are good choices because they cover over the entire surface, including all joints, seams, corners, and penetrations, and conform directly to any irregular surface. This creates a fairly uniform membrane as a result, with the continuity of the barriers being achieved by the liquid nature of the product that forms itself to the substrate. However, it is not necessarily the easiest to install given that weather conditions can hamper the timing and quality of its installation. It will also require some drying or curing time before anything else can be done, and the liquid membrane material used needs to be compatible with the substrate.
Self-adhered membranes: A different choice for WRB-AB systems can be found in self-adhered sheet membranes. Since these have been common for some time in the roofing and waterproofing industries, it is not surprising that there are similar products in use for providing barriers in wall assemblies too. In the case of this barrier type, a protective paper coating may be peeled off the sticky side of the membrane, which allows it to then be directly adhered to the sheathing. As you might expect, this type of product works best on smooth, flat, continuous surfaces. Skillful attention is needed to cut and fit the self-adhered membranes to places like building corners, discontinuous edges, openings for windows and doors, etc. It should be noted, too, that there are different types of self-adhered membranes made from different materials. This is important to be aware of since self-adhered membranes are commonly certified and used as WRBs, but they may or may not be certified as an air barrier as well. If that is the case, then a separate AB material will be needed. It is also important to note that the large number of seams along the edges of the strips of the membrane make it more of a challenge since continuity needs to be achieved along all of those edges and seams.
Foam plastic insulation: Certain foam plastic insulation, particularly if it is closed-cell rigid boards, may qualify as a WRB or an AB. This might be convenient since it is commonly installed over the face of exterior sheathing to act as a thermal barrier of continuous insulation on the outside of the building. However, keep in mind that just because it is present in the wall assembly doesn’t mean it has been tested and certified as either a WRB or an AB. In some cases, the material itself may be fine, but without a proven means to address the edges and perimeter of the individual rigid insulation boards, it does not qualify as a continuous assembly. In such cases, a separate WRB and AB are still needed.
Integrated sheathing: Some gypsum sheathing manufacturers have developed and now offer the latest innovation in barrier technology, namely, incorporating both a WRB and an AB directly into a fiberglass mat-faced gypsum sheathing. This allows one engineered product to provide multiple control layers with a single installation of the exterior gypsum sheathing. As such, integrated gypsum sheathing products allow a simpler, more streamlined installation process with less labor required compared to adding separate layers for the WRB and AB. This simplicity of the system makes it ideal for use in all types of buildings, helping to assure quality and control costs.
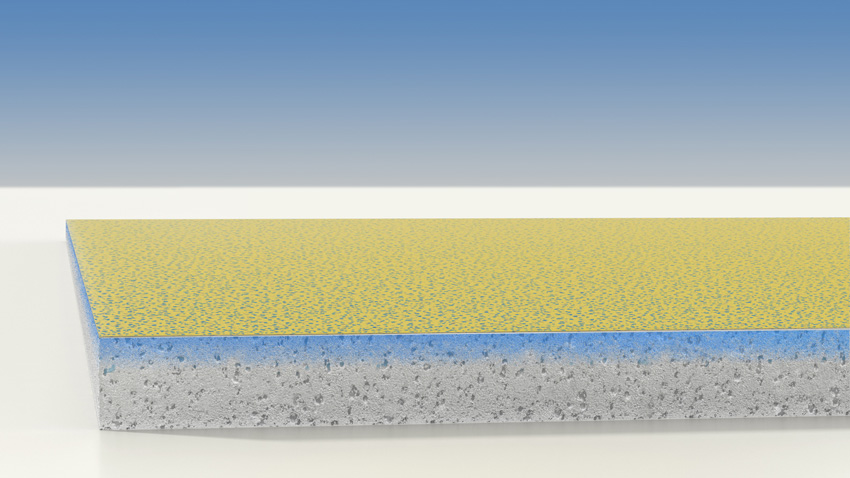
Integrated sheathing incorporates the WRB and AB directly into the sheathing in a single product, eliminating steps and reducing construction time.
Integrated sheathing systems address joints and seams in an equally simple and effective manner. Liquid flashing is applied and spread just in the areas where it is needed over fasteners, along joints and seams, and around openings. This is far simpler and much less time consuming than covering the entire face of the sheathing with a liquid membrane. Further, the combination of the integrated sheathing and the liquid sealant has been tested and certified to provide a truly continuous AB and WRB. In essence, the whole system uses the best of all the other choices—the effectiveness of proven barriers in the flat, smooth areas of a building, and the flexibility to fully address irregular shapes, surfaces, corners, edges, and other conditions.
Based on this brief review of common WRB-AB choices, it is clear that the manner of addressing continuity is different for each of them. This is an important issue because it is often that continuity that makes the difference between an effective barrier and one that will leak. The best choice will likely depend on the details of a particular building, the availability of skilled installers (or lack thereof), and the project budget.
Drying Capability
Selecting an effective WRB and AB and making them truly continuous means that bulk water and unwanted air infiltration is kept out of the wall assembly from the exterior side of the wall. However, there is a legitimate concern about moisture in the form of liquid and/or water vapor penetrating through openings in the interior drywall and/or vapor retarder and ending up in the insulated cavity of the wall assembly. If that vapor is then trapped because of the presence of a vapor barrier and not allowed to diffuse or escape from the cavity, it can cause mold to grow or moisture damage to the wall and surrounding construction. The best solution is to use a WRB and an AB that can restrict bulk water and air but still be permeable enough to allow vapor to diffuse out over time, thus creating a full drying capability for the wall. This aspect of an exterior wall assembly is commonly reinforced by building scientists as a safeguard against degradation of the wall. There are a variety of products that provides this balance of WRB-AB protection and permeability to allow for cavity drying when needed, with integrated sheathing being one common example of such a product.
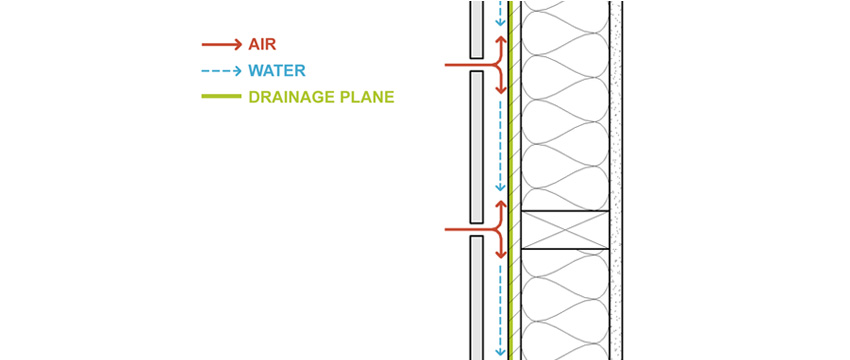
The ability of an exterior wall to drain away moisture and allow drying is critical to the success of its long-term durability and performance.
Regardless of which choice is selected for AB and WRBs, some form of cladding is typically installed over it to create the exposed, visible, exterior wall surface. Such cladding could be masonry, metal, wood, fiber cement, or some other material, any or all of which could be used on a single building. Each of them will have their own performance and design characteristics, and their manufacturers will have their own recommended or preferred method of installing them. In virtually all cases though, good building science indicates that the key to a successful wall assembly is to provide for a gap or space between the selected cladding and the WRB-AB. This gap allows for any bulk water that does enter behind the cladding, or any condensation from moisture that occurs, to drain down the face of the WRB and exit the assembly safely through flashing, weep holes, etc. This makes the wall “drainable” but also helps directly with the drying capability of the wall since the gap and related drainage outlets will allow moisture in the wall assembly to escape harmlessly.
Construction Technique
Understanding the principles discussed so far is important, but often the biggest issue in achieving them is to understand the capabilities and limitations of a specific construction technique. For example, providing a gap and drainage space has been very commonly and appropriately used in masonry construction to retain the integrity of the total wall assembly. This approach recognizes that some masonry may be porous and allow for bulk water to enter behind the masonry cladding, or rainwater may enter somewhere else (such as near an opening) and get trapped between the masonry and the sheathing. Hence, common flashing techniques and weep holes or similar means are employed to collect and drain away the water.
For cladding types other than masonry, such as panels made of metal, composite, wood, or other materials, the cladding may be supported and held away from the sheathing with some type of support or spacer system. Commonly, metal girts or spacers are used that can be installed in various ways and with different components or coordinated systems. Horizontal metal Z-girts are one common type of this construction technique to create the gap and keep everything else in place. The horizontal girts are secured to the framing members and, in turn, provide continuous support of the cladding secured directly to the girts. The Z shape provides a space for rigid insulation to be installed in the depth of the girts and supported in place. Note that these Z-girts are often installed either horizontally or vertically and the horizontal installation may interfere with drainage capabilities across the sheathing surface. In those cases, if not properly detailed and accounted for, the top face of the girts could collect water.
Spaced clips are an alternative approach to using Z-girts. These individual clips are fastened through the sheathing into the framing in order to be securely held in place. The clips then provide spot support for cladding instead of the continuous support provided by girts. Similar to girts, the depth of the clips can be sized to provide the appropriate thickness of rigid insulation to be installed between the cladding and the sheathing. Unlike horizontal girts, the clips maintain space between them to allow for the free drainage of water down the sheathing/WRB surface.
Cladding Impact on WRB-AB
A key component of any of these construction techniques discussed is the use of fasteners, such as screws or bolts, to secure cladding directly to the exterior sheathing or secure girts, spacers, and insulation that support the cladding. In so doing, these fasteners must also penetrate through the WRB-AB that was so carefully prepared to be continuous. In so doing, they breach the WRB-AB and can cause leakage unless they are properly addressed. Hence, the various means of providing cladding attachment will have a direct impact on the integrity and continuity of the WRB-AB on the exterior sheathing.
All of these different principles and techniques that we have touched on so far lead us to the question of exactly how much the attachment of cladding impacts the continuity or creation of leaks in WRBs and ABs. Some would hypothesize it is a big impact, some might declare that the impact is minor, and others might say that “it depends” due to the variables and choices described. In order to fully determine who the real “culprit” is in WRB-AB leakage, we need to ask some fundamental questions:
- How significant are the fasteners when they penetrate?
- How significant are the cladding attachment systems and their orientation (vertical or horizontal)?
- Does the type of WRB-AB used in a wall assembly make a significant difference on performance?
- What building conditions and climate traits come into play?
In order to answer the questions properly, the best way to proceed is with demonstration testing of different wall conditions subjected to different air and water conditions, all in a testing laboratory.
The Test
In response to all of the foregoing, we now turn our attention to some actual demonstration testing that was undertaken specifically to address the questions we have raised. In order to understand the parameters of this work, we focus first on describing the basic physical setup of the testing and then look at the specific test methods used.
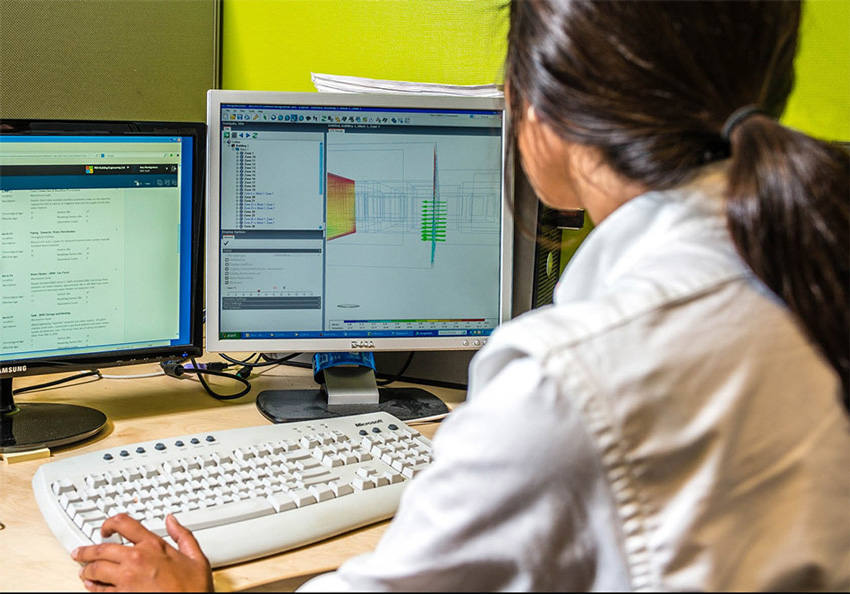
Independent testing by RDH Building Science in Waterloo, Ontario, is the basis for the test and results discussion in this course.
Test Setup
The specific test that we are looking at was carried out by RDH Building Science in Waterloo, Ontario, in 2017. RDH is a consulting, research, and forensics firm that focuses on helping clients make informed decisions that lead to durable and effective buildings. RDH Building Science Laboratories contributes to that goal by providing high-quality research and development services. The testing that was performed was based on ASTM E331-00 (2016): Standard Test Method for Water Penetration of Exterior Windows, Skylights, Doors, and Curtain Walls by Uniform Static Air Pressure Difference. This standard describes the physical means and methods required to conduct the test and then provides the required air and water pressure levels and amount of time for which each tested wall sample must be subjected.
RDH constructed six test wall panels, 4 feet wide by 8 feet tall, as the basis for the testing. All six of the test panels were representative of commonly used systems that are code-compliant for typical commercial buildings using metal framing with exterior gypsum board sheathing. Five of the panels focused on different means of providing cladding support with different fastening approaches. Across the six panels, three different WRB systems were employed: athin-mil fluid-applied WRB, a thick-mil fluid-applied WRB, and integrated sheathing.
In order to address the different cladding attachment approaches, two different metal Z-girt arrangements were tested: flange down, which is the proper and recommended method, and flange up, which is commonly seen in the field. This arrangement was tested to see if it made any actual difference in the integrity of the WRB. This arrangement may also increase the risk of liquid water accumulating on the face of the exterior sheathing. In addition to Z-girts, two different cladding attachment clip types were included in the test setup: spacers made with a fiberglass material with lower thermal transmission rates than pure metal, and thermally broken metal spacers. These variations provided a full range of common construction techniques in the interest of providing the most relevant data.
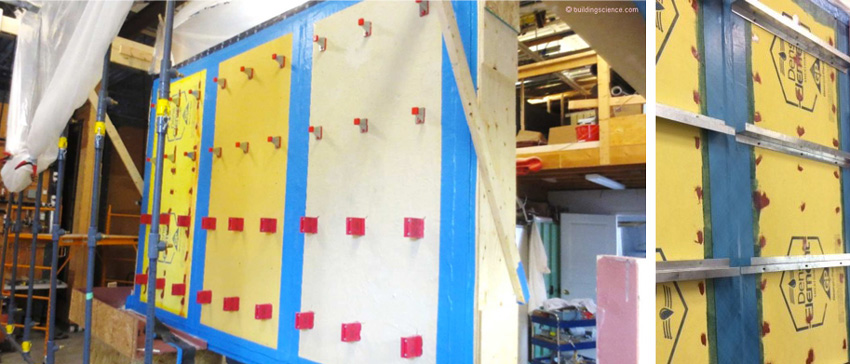
The testing methodology included different types of spacers and Z-girts in differing configurations constructed as part of the six different test panels, each of which were 4 by 8 feet in size.
In addition to the girts and clips, one of the 4-by-8-ft test panels was constructed using a layer of 2-inch-thick XPS insulation board applied directly over the exterior sheathing. The insulation was attached using 4-inch-long, self-drilling, self-tapping screws with large (1-inch-diameter) plastic cap washers. For testing purposes, the top edge of the insulation was cut to bevel backward toward the wall, forcing some water behind the insulation.
Note that none of the 4-by-8-ft test panels contained any other locations for water to penetrate other than cladding support connections. By design, there were intentionally no windows, pipe penetrations, joints, material transitions, or other potential water-entry locations. This kept the focus of the testing purely on the cladding attachment system over the WRB/sheathing without introducing the additional variables of wall openings or other penetrations.
In order to test differing installation techniques commonly seen in field construction, two cladding attachment methods were used to secure the clips and Z-girts. The first group was installed tightly against the sheathing or WRB surface, and the second group was spaced away from the sheathing or surface with different spacer thicknesses to allow water to flow behind the attachment device. The rigid insulation board was installed in a similar manner. One section was installed tight to the sheathing, and two other sections were spaced away from the sheathing at different spacing thicknesses to create a gap between the back of the insulation and exterior face of the sheathing.
Test Methods
With all of the panels constructed, set up, and in place, water-spray nozzles were then positioned on supports all facing the test wall sections and all in accordance with the protocols and specifications of ASTM E331. The intent of the spray nozzles is to simulate rain conditions at different intensities as controlled by the laboratory personnel and following the parameters of ASTM E331. In order to test for wind conditions, negative air pressure was created on one side of the wall and positive air pressure on the opposite side, again all in accordance with ASTM E331 protocols. Note that these water and wind protocols establish set conditions and amounts of time that the wall panels need to be subjected to those conditions. The building codes then determine the minimum level of performance that a wall needs to meet in order to be compliant. Since the intent of the testing was to take the wall assemblies to the point of failure (i.e., find out what it takes for them to leak), the levels of water and air pressure were incrementally increased up to and then beyond the code requirements. Nonetheless, all of these conditions were administered appropriately in accordance with ASTM E331 methodology.
For testing the water resistance of the wall panels, code-compliant water-resistance testing levels require a continuous spray of 5 gallons per hour per square foot for a period of 15 minutes. The actual water application rate during the testing was higher at 7.5 gallons per hour per square foot and longer at a full 60 minutes. The same water-spray application was repeated for an additional 60 minutes for each of the five different air-pressure conditions (i.e., 5 hours of total testing instead of 15 minutes).
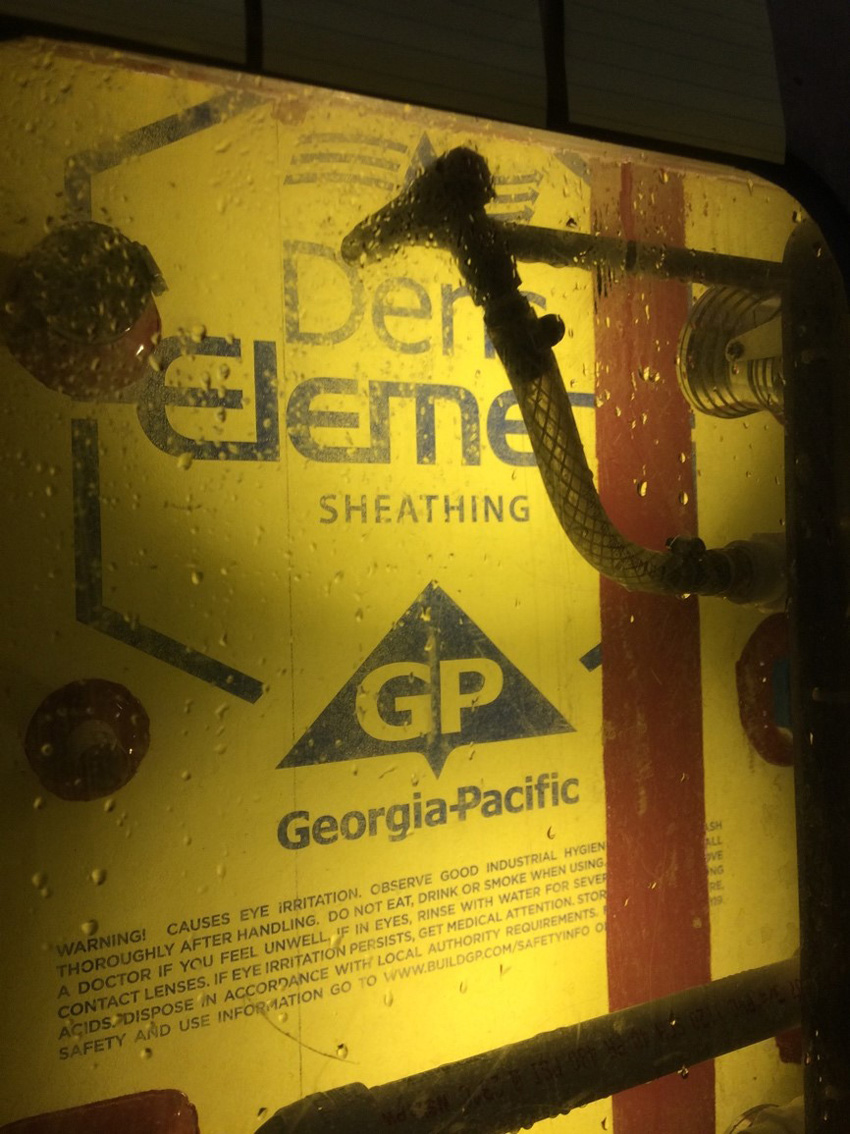
The test panels were subjected to water-spray nozzles and air-pressure differences according to ASTM E331 protocols to simulate code minimum conditions and more extreme weather conditions to determine the points of failure.
Similarly, air pressure requirements for code compliance call for a single pressure difference of 140 pascals (Pa) between inside and outside (equivalent to a sustained wind of approximately 25 miles per hour). The testing actually included five other air pressure conditions:
- 0 Pa: equivalent to calm wind conditions;
- 300 Pa: equivalent to sustained winds of 55 miles per hour;
- 600 Pa: equivalent to sustained winds of 71 miles per hour;
- 900 Pa: equivalent to sustained winds of 85 miles per hour; and
- 1250 Pa: equivalent to sustained winds of 100 miles per hour.
With this all-encompassing test methodology, RDH hoped to learn where the weaknesses lie in different types of wall assembly systems and under what air and water conditions.
The Test Results
After all of the test panels were set up the testing was administered as described, technicians recorded the time, conditions, and other observations throughout the test period. What follows is a summary of their findings from these tests. They answer the basic question of how each of the different panels and configurations perform under the stress of the different test conditions.
Weather Conditions
The first finding was that under normal weather conditions (i.e., the code-required level of water spray and wind/ air pressure), water did not penetrate into the wall cavity through the cladding attachment fasteners in any of the assemblies. This is fairly good news and suggests that any of the approaches described in the test panels meet code minimum requirements and need not use water leakage as a deterrent for their selection under normal conditions. However, as the testing continued and the degree of water and wind were increased beyond normal conditions, all of the test panels ultimately leaked (i.e., reached failure) as intended due to the increasing intensity of the water and air pressure conditions created. This suggests that for buildings located in areas where higher wind and water conditions are a concern, the specifics of the sheathing, WRB-AB, and cladding attachment system need to be looked at more closely.
Type of WRB
Under the controlled laboratory conditions, the WRBs were all installed properly over a small area so they would be expected to perform as intended without any complications. And indeed, the RDH team determined that the type of WRB used did not appear to make a measurable difference in the results during the testing. However, the testing showed that, in extreme weather conditions, leakage can occur when cladding attachment systems use fasteners that penetrate the WRB-AB regardless of the WRB-AB solution. RDH noted that once water found a penetration point, it followed that penetration into the assembly whether thin-mil fluid-applied WRB, thick-mil fluid-applied WRB, or integrated sheathing was the subject of the test panel.
Means of Attachment
This was observed to have the most bearing on why the assemblies leaked when subjected to the test conditions. In general, if the cladding attachment (Z-girts, spacers) and fasteners were installed tight to the sheathing or fluid-applied WRB surface, water did not penetrate around the fasteners, and assemblies did not leak until extreme conditions were reached. Likewise, if the cladding fastener was installed so it was adequately spaced away from the sheathing surface, allowing the water to drain behind the cladding attachment, the assemblies did not leak until extreme conditions were reached. Most of the leaks happened when the space between the WRB surface and cladding attachment was just enough for water to enter behind the attachment but not wide enough for the water to effectively drain.
Drainage and Drying
Since the test results determined that leakage potential exists for any type of WRB system under certain, albeit somewhat extreme conditions, there is a demonstrated need to adequately address drainage and drying potential in the exterior walls. That means the gap and drainage capability of the exterior wall remains critically important. It also means that vapor diffusion and drying potential needs to be considered and taken into account in exterior wall design, particularly in areas prone to severe weather.
Overall, the testing revealed that the real culprit in leakage through WRBs-ABs is not a failure in the WRB-AB technology itself but in the attachment systems and fasteners used to attach the cladding to the wall. In all of the conditions tested, leakage occurred when water and air at elevated levels were able to reach and travel along the attachment fasteners, through the barriers, and into the wall assembly.
Best Practices
RDH went a step further beyond the testing it performed by combining it with its general knowledge and experience on exterior wall designs to identify some best practices in the creation of high-performing exterior walls. The focus of these best practices remains on framed walls with exterior gypsum sheathing with an integrated or liquid-applied WRB-AB. Essentially, RDH bases these best-practice recommendations first on identifying a variety of risk factors and then on the appropriate responses to the particular combination of those risk factors.
Risk Factors
The risk factors identified by RDH fall into essentially three categories: climate, design, and construction factors. Climate risk factors include the specific climate zone where the building is located. Simply put, the risk of water leakage in rainy Seattle is not the same as in dry Phoenix. For purposes of their classification, the eight climate zones are broken into three types: dry (like Phoenix), coastal (like Seattle or other places on oceans or significant bodies of water), and mixed (sometimes wet, sometimes dry across the year).
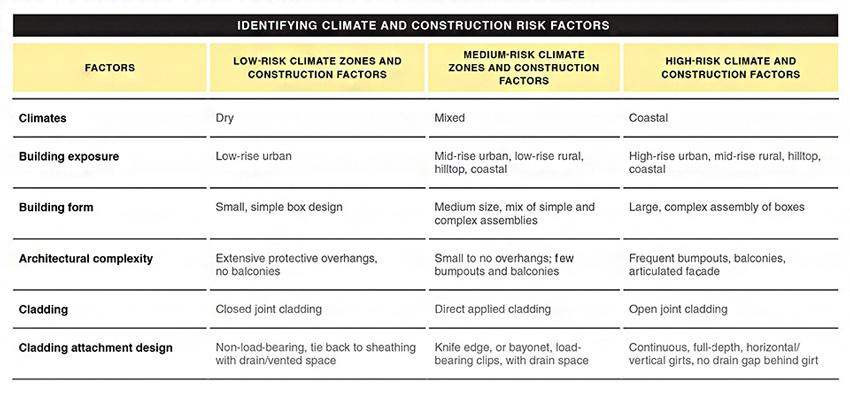
In the interest of determining best practices, RDH identified different climate and construction risk factors and defined low-, medium-, and high-risk conditions.
Design risk factors relate directly to the final design of the building. The building exposure may be predetermined by the site in terms of urban, rural, or hilltop settings, but the building height may be controllable by design—the taller the design, typically the more exposure to wind and weather if there is nothing around it to act as a buffer and protect the walls. The building form is another design factor that can influence risk. Essentially, the more complex the building form, the more likely it is that the WRB-AB and cladding systems can be difficult to install and achieve true continuity. Beyond form, other aspects of architectural complexity can influence the performance of exterior walls. These include the presence (or absence) of things like overhangs, balconies, bumpouts, and other facade articulations.
Construction risk factors are linked to the design and installation techniques of the cladding. Some cladding is intended to be closed or continuous along all of the joints (such as masonry), some is open around joints and sections (as in a rainscreen design), and some may be directly applied to the sheathing (as in siding). The method of attaching the cladding to the building is similarly a construction risk factor. The presence or absence of a drainage gap is clearly a significant factor, but so is the choice of cladding support (i.e., clips or girts).
With each of these risk factors identified, the next step is to categorize appropriate combinations of climate, design, and construction risks into a ranking order, which RDH has done and described as follows.
Low-Risk Climate and Construction Factors
RDH identifies a combination of conditions as “low risk” for leakage if all of the climate, design, and construction risk factors are low. This would apply to buildings that are first located in a dry climate. The building itself would be a low-rise structure and located in an urban setting (i.e., protected by other buildings). The building form would be small and simple with architectural features that include overhangs for wall protection but no balconies that can interrupt those wall systems. The low-risk condition is also characterized by cladding that uses closed joints (i.e., not open rainscreen type). The cladding attachment design is non-load bearing, tied back to the sheathing, and provides a good drain/vent space.
In low-risk conditions, the recommended best practice is based on simply following the instructions of the WRB-AB manufacturer. It is reasoned that this should suffice since most of these recommendations are based on meeting code requirements that are indicative of typical or low-risk wind and rain conditions. WRB-AB systems and cladding attachments were shown to perform well under such normal conditions during testing, provided the girts or any attachment plates or were screwed tight to the sheathing. Therefore, tightly screwing everything down is presumed as an obvious best practice.
Medium-Risk Climate and Construction Factors
RDH goes on to identify a different combination of conditions as “medium risk” for leakage. This would apply to buildings located in a mixed climate with a combination of wet and dry conditions. The building itself may be a mid-rise structure in an urban setting; otherwise it would be a low-rise building in a rural setting, on a hilltop, or in a coastal location. The building form would be of medium size with a mix of simple and complex assemblies. The architectural complexity would be of a medium level with features that might include smaller overhangs and only a few bumpouts or balconies. This medium-risk condition is also characterized by cladding that is directly applied to the sheathing with no open joints. The cladding attachment design might include things like knife edge or bayonet fasteners or load-bearing clips, but in all cases, the critical drain space would need to be present.
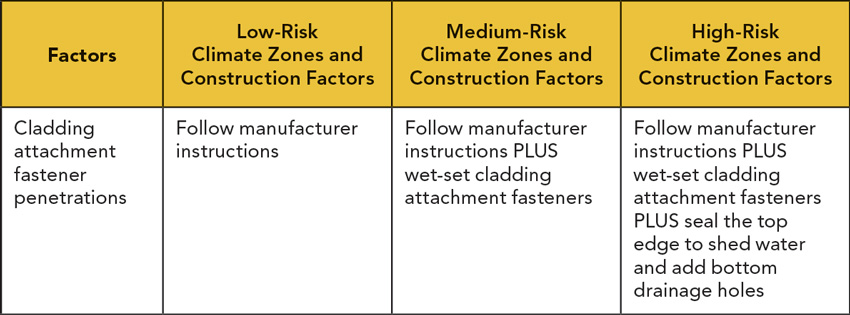
Recommended best practices for sealing against air and water leakage will differ depending on the climate and construction factors being designated low, medium, or high risk.
For medium-risk conditions the best-practice recommendations build on the low-risk recommendations in that manufacturer instructions should still be followed and all screw heads, girts, and any attachment plates still need to be screwed tight to the sheathing. However, to guard against possible leakage at this level of exposure, RDH further recommends using a wet-set sealant around all cladding attachment fasteners. That would include installing any clips or fasteners through a dab of liquid flashing or liquid-applied membrane. This is a fairly easy thing to do but does require a bit of diligence to see that it is in fact carried out at all fastener locations.
High-Risk Climate and Construction Factors
Finally, RDH identifies a combination of conditions that its has determined to be “high risk” for leakage. This would include buildings located primarily in a coastal climate where wind and rain conditions are known to be more continuous or intense. The building itself would be a high-rise structure in an urban setting or a mid-rise project in a rural setting. It could also include any building on a hilltop or located immediately on a coast or shore. The building form would be a large, complex assembly of box shapes, meaning many outer and inner corners that could compromise the integrity of the WRB-AB. The architectural features could include frequent bumpouts, balconies, or an articulated facade. The high-risk condition is also characterized by cladding that is open jointed as in a rainscreen type of facade. Cladding attachment would be done using continuous, full-depth girts that are installed either horizontally or vertically but with no drain gap behind the girts (held tight to the sheathing).
For high-risk conditions, RDH intends that everything recommended for low- and medium-risk conditions is followed during installation (i.e., everything screwed tightly, follow manufacturer’s recommendations, seal cladding fasteners). In addition, specifically when Z-girts or spacer clips are used to support the cladding, the top edges of those girts or spacers should be sealed continuously with a liquid sealant or liquid flashing. Further, bottom drainage holes should be provided in each girt so water does not collect and pool on the girts and seep into the wall assembly.
Note that all of these best-practice recommendations relate to a variety of differing factors, so they all need to be taken into account individually and holistically when deciding whether or not to implement additional steps. When in doubt, particularly if a given building overlaps these three different risk levels in certain characteristics, the conservative approach would be to do more, not less, to protect against leakage.
Conclusions
The principles of creating high-performance exterior framed walls center on maintaining the continuity of WRBs and ABs over exterior sheathing. The testing procedures and results presented in this course are meant to inform architects and other design professionals on determining appropriate measures to take to help ensure continuity and avoid leakage under differing conditions of risk. Some of the primary conclusions include the following.
Conclusion No. 1: Fasteners in Cladding Support Systems Can Leak in Heavy Weather Events
Testing has identified the real culprits in leakage is through fasteners that support cladding systems. While this was observed primarily in simulated weather events, those leaks have the potential to occur anytime.
Conclusion No. 2: Follow Best Practices Based on Building and Climate Conditions
Incorporating the best practices learned from this testing and known building science will help assure better performance of the WRB-AB in exterior walls. Remember the level of risk of leakage is based on different climate and construction factors as discussed, so discerning the conditions is the first step in determining the best practice strategies to follow.
Conclusion No. 3: Employ WRB and AB Strategies that Provide Drainable and Dry-able Wall Assemblies
In addition to purely considering WRB and AB membranes, provide construction techniques that address drying potential and vapor diffusion/permeability in the walls. This includes selecting a permeable sheathing/WRB-AB solution that will allow for proper drying of the wall if water does penetrate into the assembly for any reason.
Incorporating these conclusions into the design and construction of buildings will help improve the performance of exterior wall systems that use conventional framing and exterior sheathing with an integrated or liquid-applied WRB-AB.
Peter J. Arsenault, FAIA, NCARB, LEED AP, is a nationally known architect, consultant, continuing education presenter, and prolific author advancing building performance through better design. www.pjaarch.com, www.linkedin.com/in/pjaarch