Sustainability and Structural Steel: A Closer Look
Continuing Education
Use the following learning objectives to focus your study while reading this month’s Continuing Education article.
Learning Objectives - After reading this article, you will be able to:
- List numerous sustainable characteristics of structural steel.
- Establish an appropriate methodology for comparing the environmental impacts of structural framing materials.
- Examine the cradle-to-cradle supply chain for domestically produced and fabricated structural steel.
- Identify strategies for minimizing the environmental impacts associated with structural steel.
- Locate available resources for additional information on structural steel sustainability.
This webinar is part of the Steel Academy
While commonly recognized for its high recycled content, structural steel is a multi-attribute building material, the use of which can significantly reduce the environmental footprint of a building.
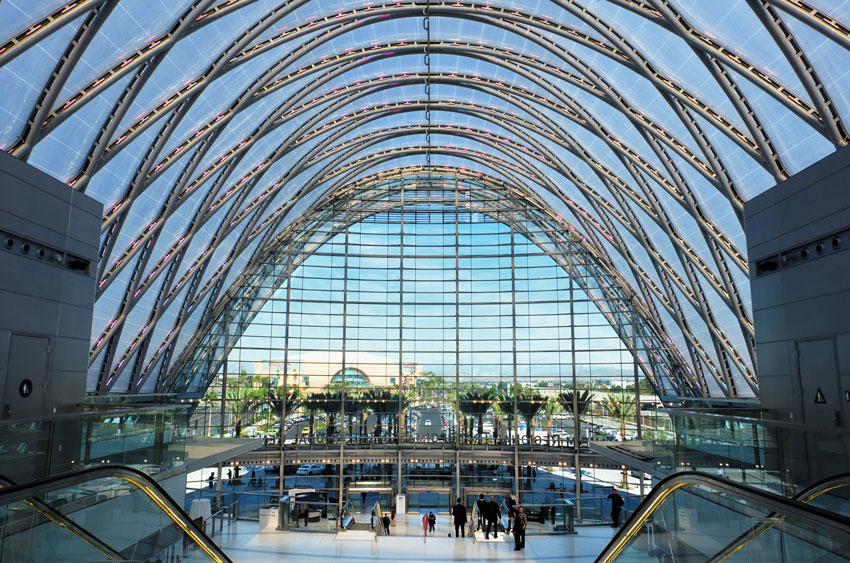
Photo courtesy of Thornton Tomasetti, Inc.
Long spans, curved steel members, and abundant natural light highlight the new Anaheim Regional Transportation Intermodal Center in Anaheim, Calif., which won a 2015 AISC IDEAS2 Award and achieved LEED Platinum certification.
The steel industry in the United States recycles more tons of waste than any other domestic industry, with 81 percent of all steel products being recycled back into new steel products. Of all steel products, an impressive 98 percent of all structural steel is actively recovered and recycled or reused. The result is that the recycled content of domestically produced and fabricated structural steel used for structural framing systems is greater than 93 percent.
“The production of steel from recycled material can use less than one-third the amount of energy needed from starting with raw ore only,” states Donald W Davies, PE, SE, president, Magnusson Klemencic Associates, Seattle. “It’s huge when buildings can take advantage of this type of recycling.”
Steel’s recyclable attributes are only part of the story, as there is a larger storehouse of sustainability benefits provided by structural steel. Today we know that steel is much more than a single-attribute material, and we recognize the many attributes of steel that contribute to sustainable construction in many ways.
Consider the following:
- Domestic structural steel mill capacity exceeds current and foreseeable domestic demand, with more than 75 percent of current demand being met by domestic producers.
- Hot-rolled structural steel mills in the United States do not use iron ore, coke, or limestone as primary feedstock materials; no mining operations are required.
- Unlike the legacy steel mills of the early 20th century, today’s structural steel mills have highly sophisticated systems to minimize emissions. They are highly automated, environmentally conscious neighbors in the communities where they are located.
- Steel does not lose any of its metallurgical properties when recycled. Consequently, the quality and properties of recycled steel are the same as virgin steel.
- Iron is a nondepletable resource, as all steel can be recycled, and any increase in demand beyond the available supply of scrap can be met by the earth’s abundant supply of iron, which comprises 35 percent of the earth’s mass.
- Structural steel mills recycle all of the water they use through a closed-loop recycling system. Less than 70 gallons of water is consumed per ton of steel produced.
- There are close to 2,000 steel fabricating firms located throughout the United States. that detail, cut, drill, bolt, and weld structural steel for building projects, providing local employment and economic stimulus.
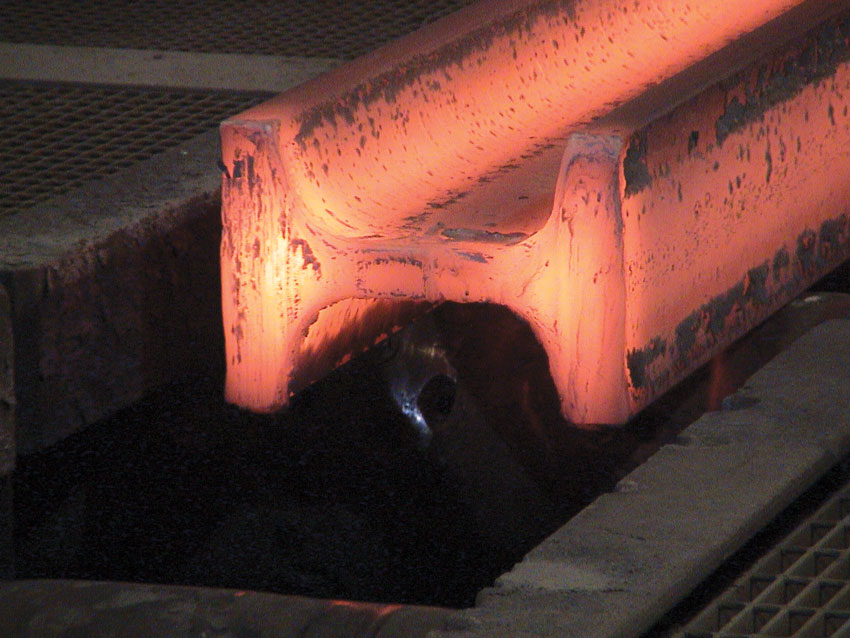
Photo courtesy of Nucor
This beam blank is exiting the caster prior to reheating and rolling into a structural section.
Comparing Structural Steel to Concrecte and Wood
In comparing structural steel to concrete and wood, Rob Still, PE, senior structural engineer at T.Y. Lin International, Lindbergh, Charleston, South Carolina, points out that wood is subject to mold, mildew, rot, and termite damage, so while it may be considered sustainable, its life expectancy is generally less than steel or concrete structures.
Compared to concrete, structural steel is a lighter, stronger material, allowing for dimensionally smaller columns, longer spans, greater openness, and reduced foundation requirements.
Of the major framing materials, only structural steel is regularly recycled back into new structural framing material. Some concrete members are down-cycled into road base. The rest goes into landfills, like wood, which cannot be recycled. Structural steel members can also be reused in place or in new building projects.
“The steel advantage really comes from its light weight, speed and ease of installation, and resistance to corrosion and deterioration,” summarizes Still.
Furthermore, the steel industry is actively working on new technologies and materials, such as buckling restrained brace frame systems and high-strength steels, to further lighten steel structures. “Both of these advancements are leading to lighter, more efficient, and more sustainable structures,” he adds.
Pointing out another advantage of steel over wood, Alison Kung-Kellerer, LEED AP BD+C, associate, Carrier Johnson + CULTURE, San Diego, notes that steel is noncombustible with a high melting point, making it fire resistant and able to be exposed to view. “Wood, on the other hand, most often has to be covered with gypsum wallboard or plaster to be fire resistant,” she explains. “Steel is also ductile, rather than brittle, so it can be used more efficiently than concrete when considering seismic forces.”
Steel framing also offers the most long-term flexibility in that it easily can be strengthened in the field by welding on stiffening elements should loading needs change over the life of a building, she says.
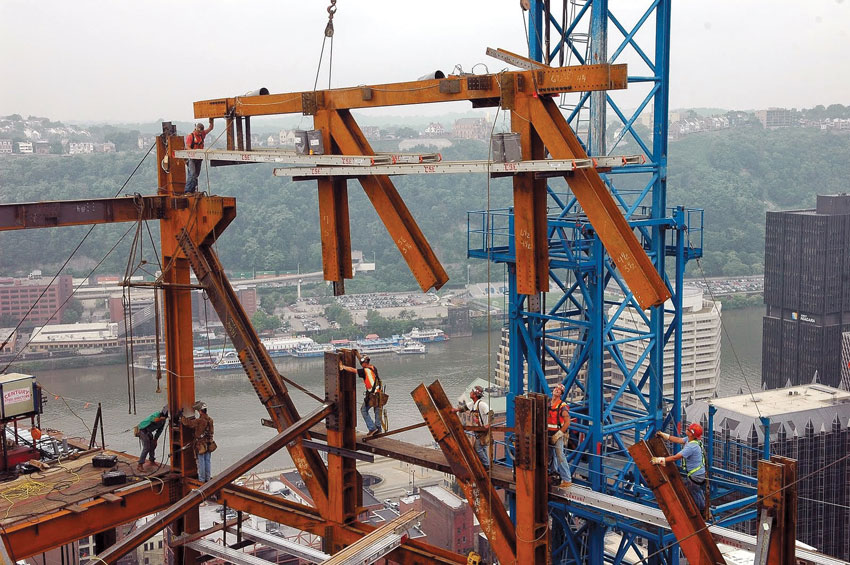
Photo courtesy of Sippel Steel Fab
Shown during the erection of the structural steel frame, the new 33-story LEED Platinum Tower at PNC Plaza sits on a five-story podium and houses more than 800,000 square feet of commercial office, auditorium, lobby, cafeteria, parking, and amenity spaces. The superstructure is entirely steel framed using composite steel beams with a steel-braced lateral system. In all, the project uses 8,000 tons of structural steel and 200,000 bolts.
Another example of the efficient use of structural steel is that it can be used for both the required support of structural loads and as a finish material, according to Tim Keil, RA, associate principal, Studio Ma, Phoenix.
He also adds that “the ability to create seamless members and exploit the material’s high strength through welding is a major benefit over other structural materials.”
In a nutshell, if building teams are looking to reduce their carbon footprint, the real secret in the sauce, says Davies, is in making building choices that stand the test of time. “Steel solutions can achieve both resiliency and adaptability for the future in ways other materials can’t on their own,” he asserts. “The steel industry has always been building hundred-year buildings.”
And it remains true as well that domestically produced structural steel is a highly recycled and recyclable material. The recycled content of domestically produced, hot-rolled structural steel is greater than 93 percent. The variation is the result of small amounts of ferrous and nonferrous materials being added during the production process to achieve the proper chemical balance for the specific grade of steel being produced.
Unlike other materials, such as concrete, which is typically down-cycled into road base, steel is 100-percent recyclable, without any loss of the metallurgical properties. Structural steel is a true cradle-to-cradle building framing material.
Of course, it’s one thing to be fully recyclable, in theory, but another to actually be recovered and recycled or reused. Industry wise, 81 percent, by weight, of all steel products are recovered, with an impressive 98 percent of structural steel recovered for recycling or reuse. This compares to aluminum’s recovery rate of 65 percent and a rate less than 40 percent for wood. Steel, by far, has the highest recovery rate.
Properly Comparing the Environmental Impacts of Structural Framing Materials
The construction industry, as a whole, consumes a significant percentage of the world’s natural resources and energy.
“As architects and designers, we believe that it is our professional responsibility to minimize the impact of the structures we design and build,” states Kevin Nasello, AIA, LEED AP BD+C, senior associate and director of sustainability, CetraRuddy, New York. “Analysis of the embodied energy and other environmental impacts associated with each material is an imperative first step to a sustainable building design.”
However, performing a true comparison is a complicated endeavor. Unfortunately, it is not uncommon for building teams to mistakenly evaluate a framing material’s sustainability characteristics based upon CO2 emissions per unit of mass alone. On the contrary, a material’s contribution to the environmental impacts of a project are a function of the amount of material used, the process utilized to make the material, and the impacts directly associated with the material. You have to adjust the mass-based numbers to account for the differences in the amounts of each material that would actually be used in the building.
“Framing materials cannot be compared directly to each other,” states John Cross, PE, LEED AP, vice president, American Institute of Steel Construction, Chicago. “Simply put, the same square footage in a building does not require equal amounts of steel, concrete, or wood. Structural steel is a stronger, more durable material, and less structural steel is required to carry the same structural load as would be required of concrete or wood.”
In order for these factors to correctly be evaluated, a more comprehensive life-cycle comparison is in order. For example, building teams must consider factors like the time to construct the building, the carbon footprint required to produce a specific quantity of material, the impact of other systems such as increased foundations in concrete buildings, and the thermal qualities of the materials, says Still.
Adding to the list, Nasello asks the following questions: How is the material extracted at its source? How it is manufactured, including use of raw materials, recycled content, and pollution, as a result? What is the method of transportation to the construction site? What are the means and methods of construction? What about on-site and off-site waste management, use, and maintenance? And if it is demolished, how is it recycled?
Taken together, Davies points out that the longest standing, most durable buildings typically have the potential for the lowest overall carbon footprints because they are correctly evaluated over time. “Those findings get even better when our building materials that are taken out of a deconstructed project are able to be reused for a next-generation design,” he adds.
As opposed to a single-attribute comparison, a whole building life-cycle assessment (LCA) produces a more objective, thoroughly evaluated comparison based upon multiple factors. A word of caution, though, as building teams might mistakenly believe that all a LCA comparison requires is a schematic design of a building, a list of the environmental impacts associated with all of the materials that will be used in the building, and a simple drop-in of the numbers into an estimation tool to create a legitimate building comparison.
“Pushing a ‘smart’ button and receiving a list of comparative environmental impacts for two building alternatives is not possible. In fact, nothing could be farther from the truth,” states Cross.
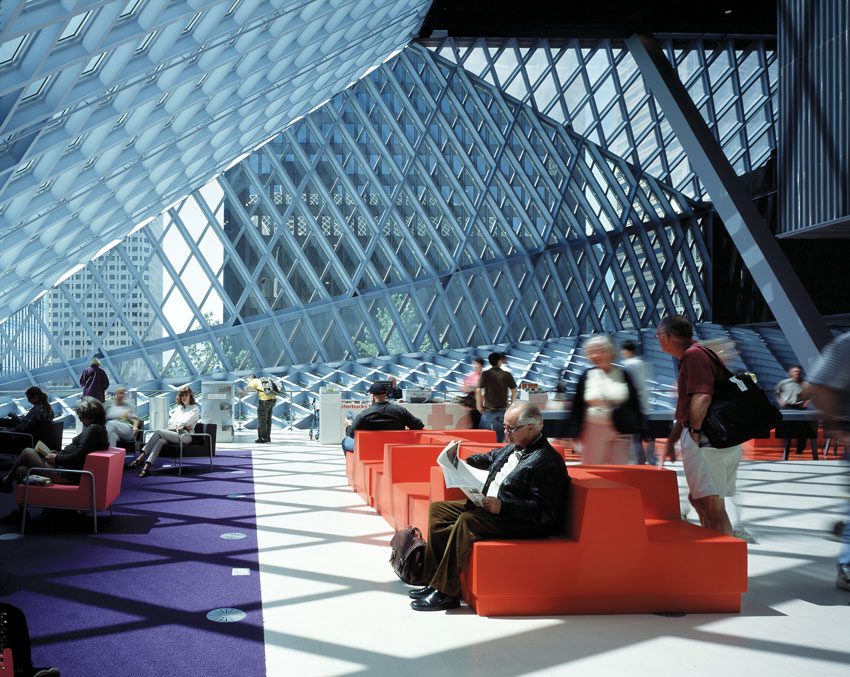
Photo courtesy of Pragnesh Parikh/MKA
At the Seattle Central Library, the design of a steel diagrid eliminates 90 percent of the aluminum that would typically go into a facade mullion system.
For a More Accurate LCA
In order to truly conduct a whole building LCA, the project team must answer the following questions:
- What portions of the building are to be considered in the analysis?
- How are the building alternatives selected?
- What is the basis of comparison between the two building alternatives—materials or design?
- At what stage of design should the comparison be performed?
- How will the quantity of materials used in the two alternative building designs be determined?
- How accurate are the material quantities being used?
- Is operating energy to be included in the evaluation?
- What was the scope of the collection of the impact inventories?
- Are all product inventories consistently using the same scope?
- What methodology and assumptions were used in determining the environmental impact inventories for each product or material?
- What is the veracity of the environmental impact inventories used for each material or product?
- What environmental impact categories will be evaluated?
- What level of environmental improvement is desired for each category?
- What level of environmental detriment will be tolerated in each category?
- How will impact categories be prioritized against one another?
While the environmental product declarations (EPDs) furnished by a growing number of building product manufacturers and industry associations can help building teams fill in some of these blanks, they are limited in their contribution toward this end.
“EPDs often measure different variables at the start and end-use stages, and it is a bit of an apples and oranges comparison,” reports Davies. A better comparison “is a long-term goal, but our industry is simply not there yet.”
As a case in point, MKA was recently commissioned for a project where the client wanted to pursue an all cross-laminated timber (CLT) design. To thoroughly vet the option, MKA created comparative options and analyzed various options, in detail. In the end, the structural engineers parametrically combined the options in optimized building choices.
“Even with the optimized mass timber frame, it was not a clear, low-carbon winner over efficiently designed composite steel or other frame alternatives, when all comparative building requirements, including acoustics and vibration, were taken into account,” he reports.
Ultimately, an efficiently designed composite steel frame showed a slightly lower total cradle-to-grave carbon footprint, but the variability between the EPD data didn’t allow for declaring any one winner on a material-only basis.
“During design, I would argue that true sustainability comparisons are not practical with today’s available material information,” he suggests. “The most sustainable solutions, though, will often come from architects and engineers using materials for what they are most efficiently suited, exposing the materials to limit secondary finishes, and finding opportunities where more than one function is achieved for each material used. Most importantly, looking for the options that give the greatest chance for the building to remain standing for the longest period of time is key.”
On that note, Christopher Alt, RA, principal, Studio Ma, Phoenix, states that building with steel typically results in more openness and transparency, taking up less space within the floor plate. Overall, this usually yields a higher net-to-gross ratio, freeing up more usable space within the same footprint. Or, conversely, it allows for smaller buildings with the same amount of usable area.
Additional LCA Recommendations
To assist building teams with performing whole building LCAs, the following key points are recommended:
- While simplified tools that estimate environmental impacts may be interesting, they should not be relied upon to accurately determine the relative environmental impacts of two alternative building designs.
- Any whole building LCA comparison must be based on structural quantities determined by a licensed design professional competent in the practice of structural engineering using an analytical design tool, not parametric estimates of material quantities generated by a simplified LCA tool.
- Just as a competent structural engineer should be determining material quantities, a competent professional skilled and experienced in the performance of whole building LCAs should perform the LCA. This task should not be assigned to a member of the design team who is unskilled in the use and interpretation of LCAs.
- At this point in the evolution of whole building LCAs, the comparison of iterative designs using similar products and materials is much more instructive, reliable, and worthwhile than attempting to compare buildings with dissimilar materials and products.
- Evaluation of building operating energy is best performed outside of the LCA by energy professionals using tools specifically designed for that level of analysis.
- Material producers and product manufacturers should be encouraged to publish environmental impact inventories for their products that clearly delineate the scope and methodology used to determine those impacts.
- Any comparison of materials, products, or combinations of materials and products into assemblies and/or the whole building should only be performed when all products and materials are using consistent scopes and methodologies.
- Rather than rely on a cookbook approach to determining the relative importance of increases and decreases in environmental impacts, the design team should evaluate a broad range of impacts in the context of global, regional, and local priorities.
The Cradle-to-Cradle Supply Chain of Structural Steel
To truly appreciate the sustainability of structural steel, it is necessary to understand the unique cradle-to-cradle life-cycle journey it takes, a journey that results in the WorldSteel Association referring to steel products as “the permanent material in the circular economy.”
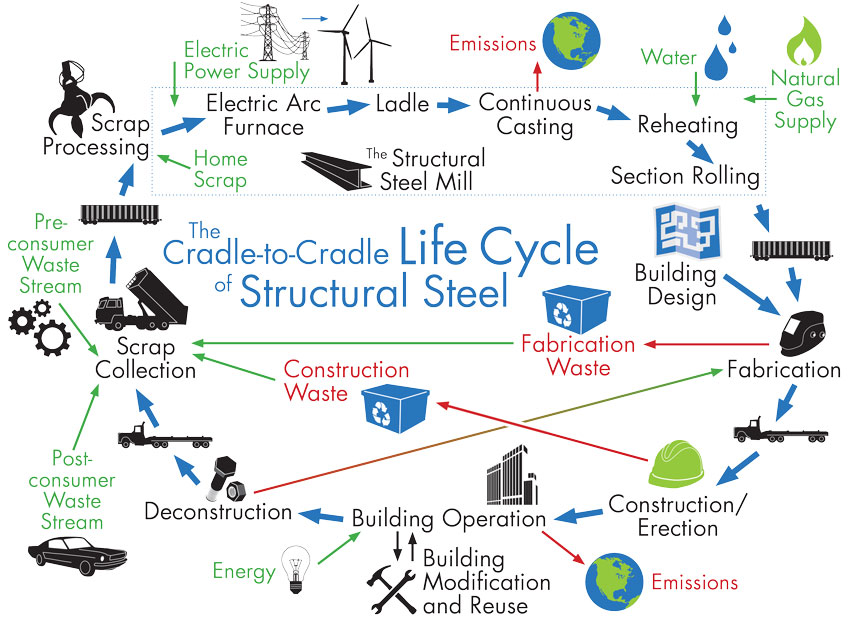
Image courtesy of American Institute of Steel Construction
Shown is the cradle-to-cradle supply chain of sustainable structural steel.
The process begins with scrap collection following by scrap processing, mill production, transportation to a steel service center/warehouse or fabricator, fabrication, transportation to the job site, erection, building operation, deconstruction, and back to scrap collection—a true closed-loop process. Scrap collection takes place from both pre- and post-consumer waste streams, with automobiles, an estimated 5 million of them in 2016, topping the list of products recycled into structural steel.
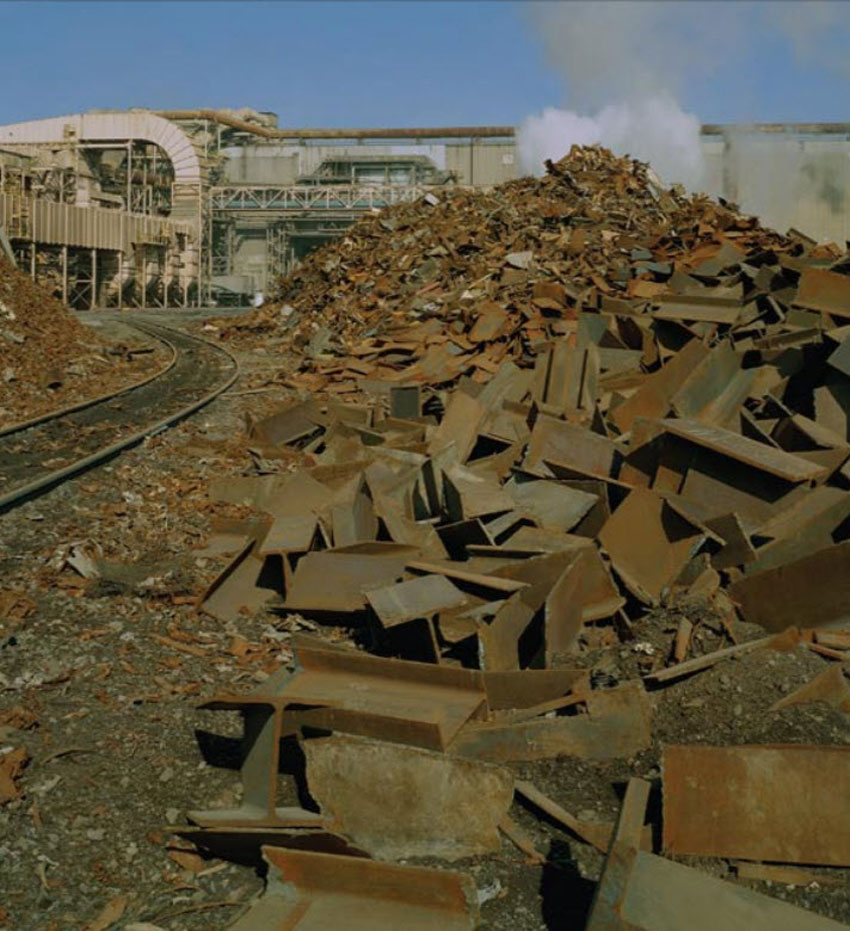
Photo courtesy of AISC
Segregated scrap grades in a scrap storage yard await recycling into structural steel.
Next, scrap processing takes place at a mill facility or remote marshalling yard. Typically, the scrap is shredded into smaller pieces, with ferrous scrap magnetically separated from other materials. The majority of scrap originates within 400 miles of a mill and is shipped to the mill by truck, barge, or rail. Once at the mill site, the scrap is stockpiled by scrap classification.
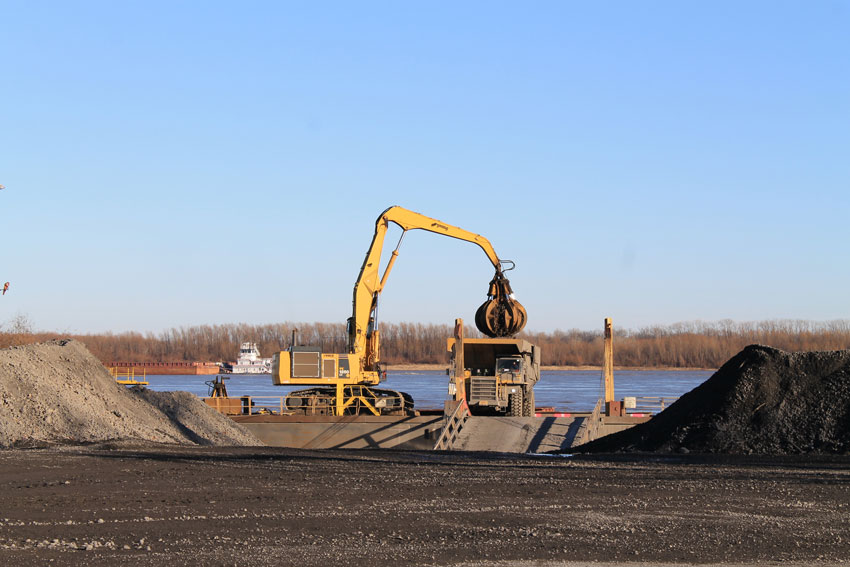
Photo courtesy of AISC
Scrap being transferred from a barge to the scrap yard at Nucor-Yamato steel’s (NYS) mill facility in Blytheville, Arkansas.
Mill production begins with placing of scrap into a charging bucket based on a prescribed “recipe” of so many multi-ton scoops of shredded automobiles, old appliances, manufacturing scrap, and demolition waste. The 100-plus-ton bucket of scrap is then discharged into an electric arc furnace, which is heated to 3,000 degrees Fahrenheit. A foamy by-product called slag floats to the surface and is skimmed off to be sold for processing into road beds, after which the remaining molten steel is transferred into a ladle. Small amounts of other elements, such as silicon, manganese, vanadium, and molybdenum, are added to create the chemistry required for the final product grade, typically A992 for hot-rolled structural steel sections. The mixture in the ladle is stirred by injecting argon gas through the mix, and after about 40 minutes, a sample is analyzed by optical spectrometry to verify the proper chemical consistency.
The molten steel is then poured from the ladle into a tank from which streams of steel flow down a long mold and form into a beam blank at 1,800 degrees Fahrenheit. The steel is then quenched with water to cool and strengthen it, with the water recaptured for later reuse. The beam blank is reheated to 2,100 degrees Fahrenheit in a natural gas-fired oven and then passed back and forth through giant rollers until the steel reaches its final shape before being cut to standard mill lengths of between 30 feet and 80 feet for shipping. This entire mill process takes approximately 3 hours from scrap selection to final cutting.
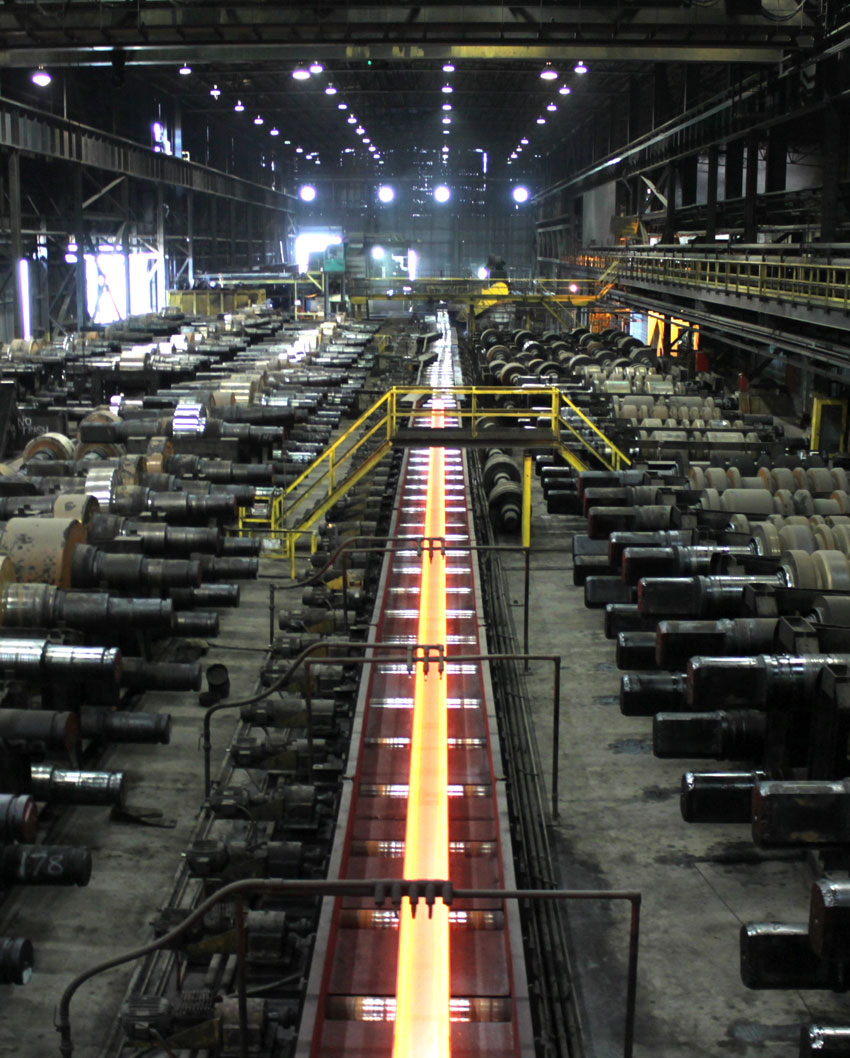
Photo courtesy of AISC
The rolling mill transforms the reheated beam blanks into precisely dimensioned structural sections.
To appreciate the extent to which structural steel production has advanced, when the Empire State Building was built in 1931, the structural steel weighed 57,000 tons with a strength of 36 ksi. Mill production of that steel required 684,000 man-hours, with 165,300 tons of CO2 emissions being produced. In contrast, today’s structural steel, for the same project, would weigh 41,000 tons with a strength of 50 ksi. Production would only require 24,600 man-hours and result in just 39,000 tons of CO2 emissions (0.96 tons of CO2x/ton of steel).
The “new” steel is then transported from the mill to a structural steel fabricator or to a service center that stocks structural steel for purchase by local steel fabricators. Thirty-four percent of domestically produced and utilized structural steel is transported by truck, 54 percent by rail, and 12 percent by barge.
A structural steel fabricator using the structural design drawings produced by a structural engineer for a specific building project generates detailed, dimensionally accurate drawings, including connection details, for each piece of structural steel.
Based upon a sequence, optimized for shop flow and project schedule, the steel members are then cut to the proper length, drilled, plasma cut or punched, fitted up with attachments for connections, and welded or bolted together in assemblies. When required, the member is cleaned and painted or galvanized.
Finally, the members are grouped in the order in which they will be erected in the field, placed on a truck for shipping, and delivered to the project site. Any waste from the fabrication process is either reused on future projects or sold to scrap collectors to be recycled.
Environmental impacts related to the process of fabricating structural steel are minimal. Waste rates in fabrication shops average just more than 7 percent of the original material quantities, with all steel scrap being sold to recyclers for conversion back into new steel products. For each ton of steel fabricated an average of 0.06 tons of CO2 equivalent is generated by the fabrication process.
With close to 2,000 steel fabrication firms throughout the United States, local sourcing is always available.
Because the structural steel has already been fabricated off-site to strict tolerances, it can be erected quickly in the field, requiring fewer workers on the job site, safer working conditions, shorter construction schedules, and reduced emissions from construction equipment. Largely connecting prefabricated components, little waste is generated on-site. Any steel waste that is generated is separated either at the source or at a scrap facility and enters the recycling process.
No maintenance is required of the structural steel, as the product life of the steel exceeds the anticipated service life of the building. Steel framing systems can be modified to address changes in building occupancy, expansion, or requirements for adaptive reuse. Steel framing also supports easy integration of mechanical systems, supporting larger window areas, low floor-to-floor heights, less building volume, and lower energy consumption.
At the end of any building’s life, it is either demolished with the waste deposited into a landfill or deconstructed, reusing and recycling as many of the building components as possible. Structural steel buildings are typically deconstructed, with all structural steel members removed and sold for reuse or recycling. Unlike concrete- and wood-framed buildings, deconstruction of a structural steel building can often occur at no cost to the building owner as the demolition contractor will offset their costs with the income gained from the sale of the steel.
Strategies for Minimizing Structural Steel's Environmental Impacts
While structural steel’s cradle-to-cradle life cycle and environmental advantages are noteworthy, the real efficiencies and sustainable-related savings come from building design optimization.
For example, “there has been some real advancement with the strategic use of higher strength structural steel,” reports Davies. “Simply using less material is always a carbon reduction winner.”
Interestingly, it takes the same energy to melt steel in an electric arc furnace, whether it is for a 36 ksi or 70 ksi end product. Higher-strength, ASTM A913 grade steel can also be welded with little to no preheat and is now available from a domestic steel mill.
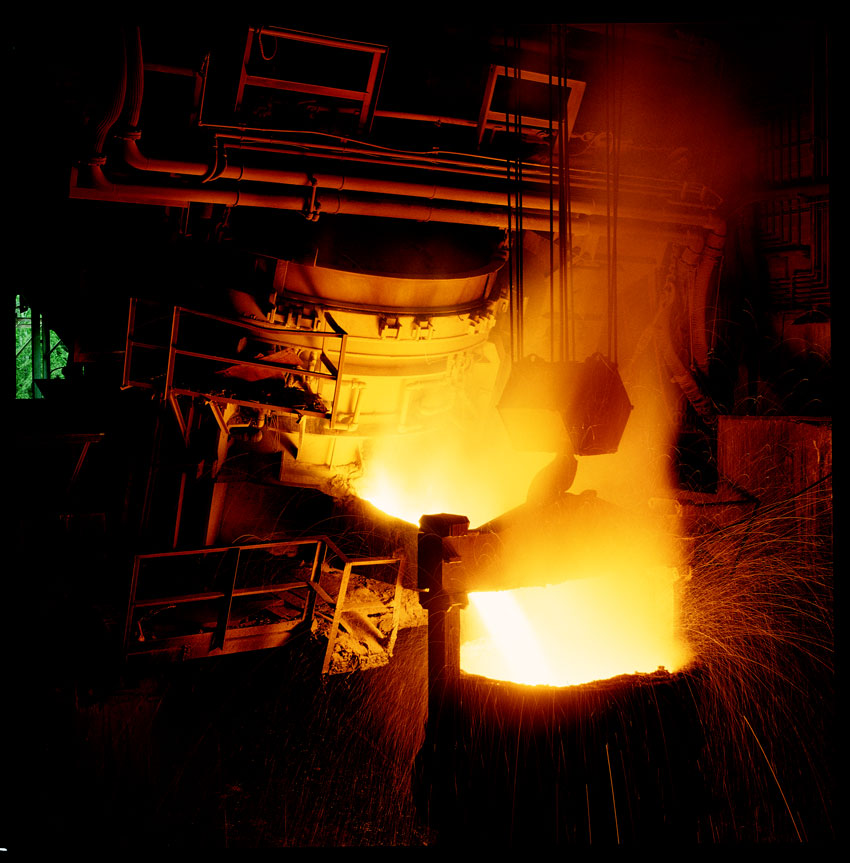
Photo courtesy of Nucor
This electric arc furnace is discharging molten steel into a ladle where alloying materials and mixing will occur.
Specifying domestically produced structural steel can also make a significant difference. Most structural steel produced overseas is not produced using the scrap-based, environmentally friendly electric arc furnace process that is standard practice in the United States. The unfortunate result of a choice to use foreign steel is to double or triple the environmental impacts associated with the steel.
Composite design, where the strength of the floor system supplements the strength of the structural steel frame through the use of shear studs, can also be used to reduce material quantities and environmental impacts.
The type of bracing system selected also impacts the amount of material used. For example, bracing in vertical planes between lines of columns provides load paths to transfer horizontal forces to ground level and provide lateral stability. Several types of frames can be used to handle a building’s lateral loads, including moment frames, chevron bracing, X-bracing, and specialty framing systems. Moment frame construction generally comes at a higher cost, but it will result in less material being used and a reduction in environmental impacts.
Because the entire length of a beam or a column in a building is not subjected to the same level of load, a smaller section containing less material may be substituted if a plate is welded to the beam or column to handle the higher level of stress in a given region of the member. While this will yield a reduction in weight, the tradeoff between weight and the increased level of fabrication requirements should be evaluated in terms of both cost and environmental impacts.
In some cases, it may be possible to use lower-weight members if the beams are cambered. If the fabricator has a camber machine in house, this is a great solution, reports Still.
It should be noted that designers often specify all structural steel to be painted with a primer prior to erection. However, this is not necessary for members that will be in the interior of the building envelope and not subject to a corrosive atmosphere generated inside the building.
Proper specification of fire protection materials can also reduce the overall environmental impacts of the framing system. Cross points out that a recent Underwriter Laboratories (UL) test, published as UL Design D982, indicates that within certain limits, the required thickness of fire protection for unrestrained floor assemblies is the same as for restrained floor assemblies. “Reflecting this in the building’s specifications effectively halves the amount of fire-protection material required for floor assemblies,” he reports.
Early Steel Fabricator Involvement
Perhaps the most important decision the project team can make to reduce the environmental impacts of the structural framing system is to engage a structural steel fabricator early in the design life of a project. The fabricator will be able to provide specific suggestions regarding the optimization of the framing system from both a material and fabrication perspective.
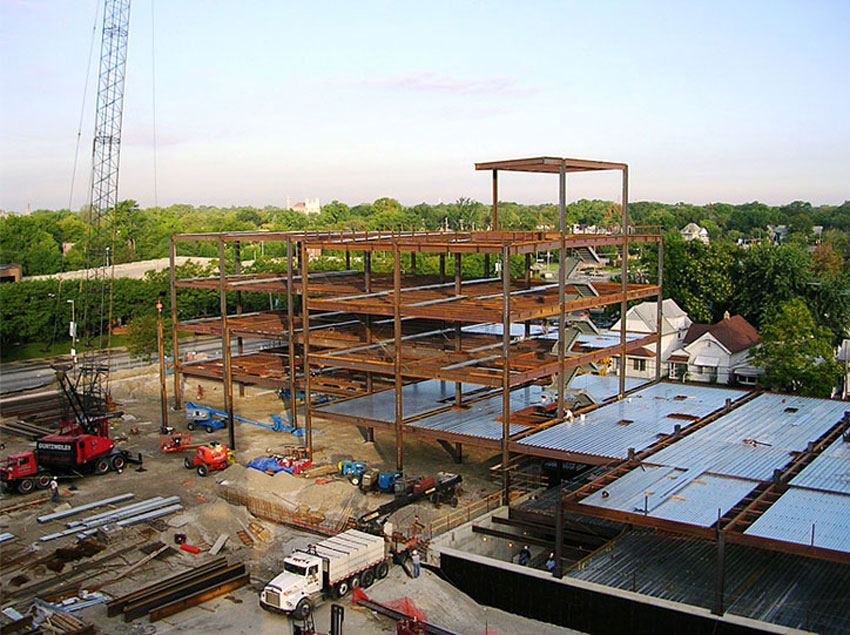
Photo courtesy of Art Iron
Pictured is the Mercy Heart and Vascular Center in St Vincent, Toledo, Ohio, under construction after the structural framing redesign included fabricator input.
The fabricator has the expertise to advise the design team on the availability of specific shapes, production locations, and methods to expedite the fabrication process.
“The selection of members that are stocked and readily available to fabricators in the project’s geographic area will save transportation impacts, the need for special rollings, and time in the overall project schedule,” explains Cross.
Still points out that there is often a lot of pressure to specify “lighter” structures. However, lighter beams often require stiffening and more complex, time-consuming, and detailed connections. The early involvement of the steel fabricator will help assess the relative tradeoffs of these design decisions.
“A thorough and complete knowledge of the systems and methods used in fabrication, erection, and assembly are needed by structural engineers to design sustainable structures,” he explains. “The engineer will often have to resist the peer pressure of making the structure as light as possible to achieve the greatest impact to a sustainable design.”
Case in Point
A recent example of where the building team successfully optimized the use of steel is the Seattle Central Library, which features a steel diagrid facade. In addition to presenting a unique, eye-catching exterior, the structural steel also serves as the facade support and the exposed lateral load resisting system, resisting wind and earthquake forces. In addition, it is part of the building’s shading strategy.
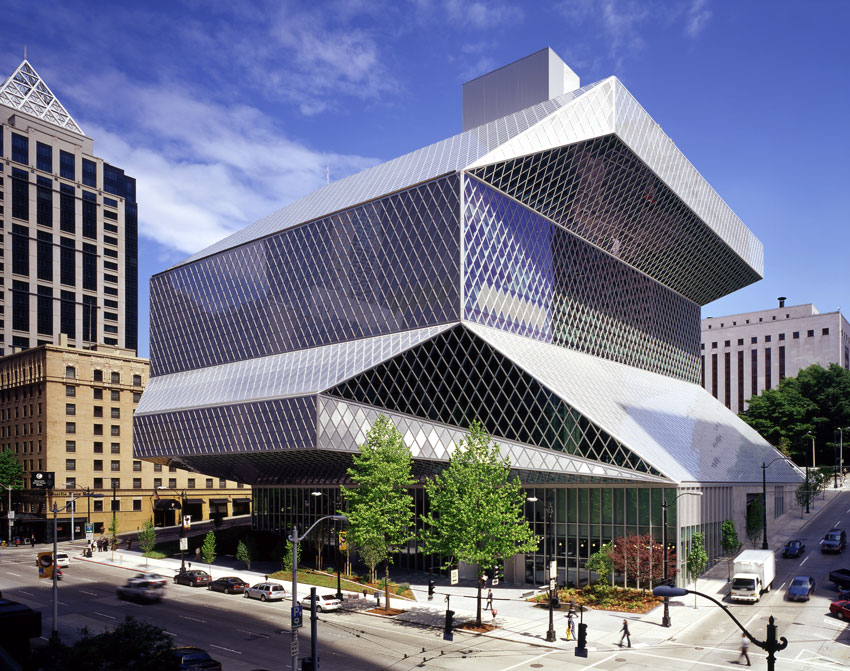
Photo courtesy of Pragnesh Parikh/MKA
For the Seattle Central Library, Magnusson Klemencic Associates designed the structural steel system to serve four purposes: the facade support, shading strategy, exposed lateral load resisting system, and an attractive aesthetic.
According to Davis, 90 percent of the aluminum that would typically go into a facade mullion system was eliminated because the steel diagrid is doing that work directly.
“It takes thoughtful efforts to achieve multifunctional goals like this, but that is where true sustainability comes into a design,” he says. “Finding the optimized multiuses of a single material and expressing it with minimal further adornment is key.”
MKA was also involved with Chicago’s Blue Cross Blue Shield tower expansion. The original design of the building anticipated a future vertical expansion, but when the decision was made to proceed with the expansion, it was discovered that the concrete core was significantly off plumb and the original planned expansion was not possible.
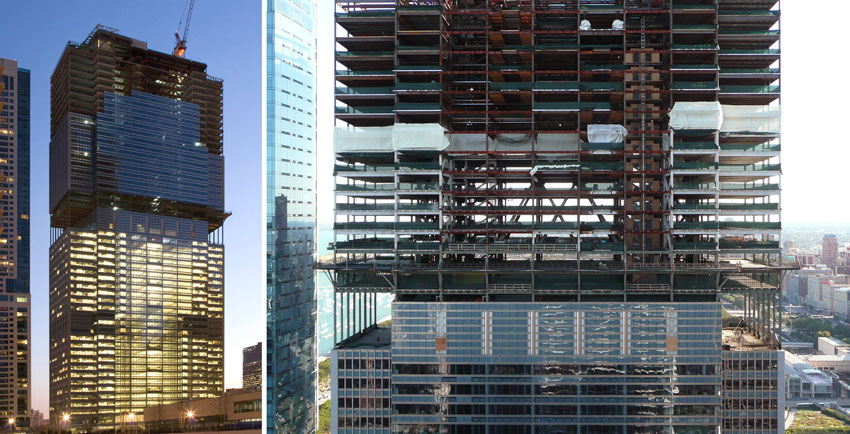
Photos courtesy of MKA
For Chicago’s Blue Cross Blue Shield’s vertical expansion, Magnusson Klemencic Associates designed new steel transfer trusses on the original roof, which was originally designed to support reserve vertical load capacity. In these construction photos, it’s possible to see the transfer trusses between the original structure and the new expansion.
Fortunately, the original design incorporated a steel frame with reserve vertical load capacity and accessible steel column connection points that allowed the use of a structural steel braced frame system for the vertical expansion that minimized loads. In designing new steel transfer trusses on the original roof, this created both an efficient transition to the tower above and an integrated MEP floor with open program spaces between the trusses, explains Davies.
Similarly, a central atrium in the original tower was thoughtfully designed for future elevators and accessible steel framing for attaching new guide rails. This essentially enabled the original building to be occupied outside the atrium during the new construction.
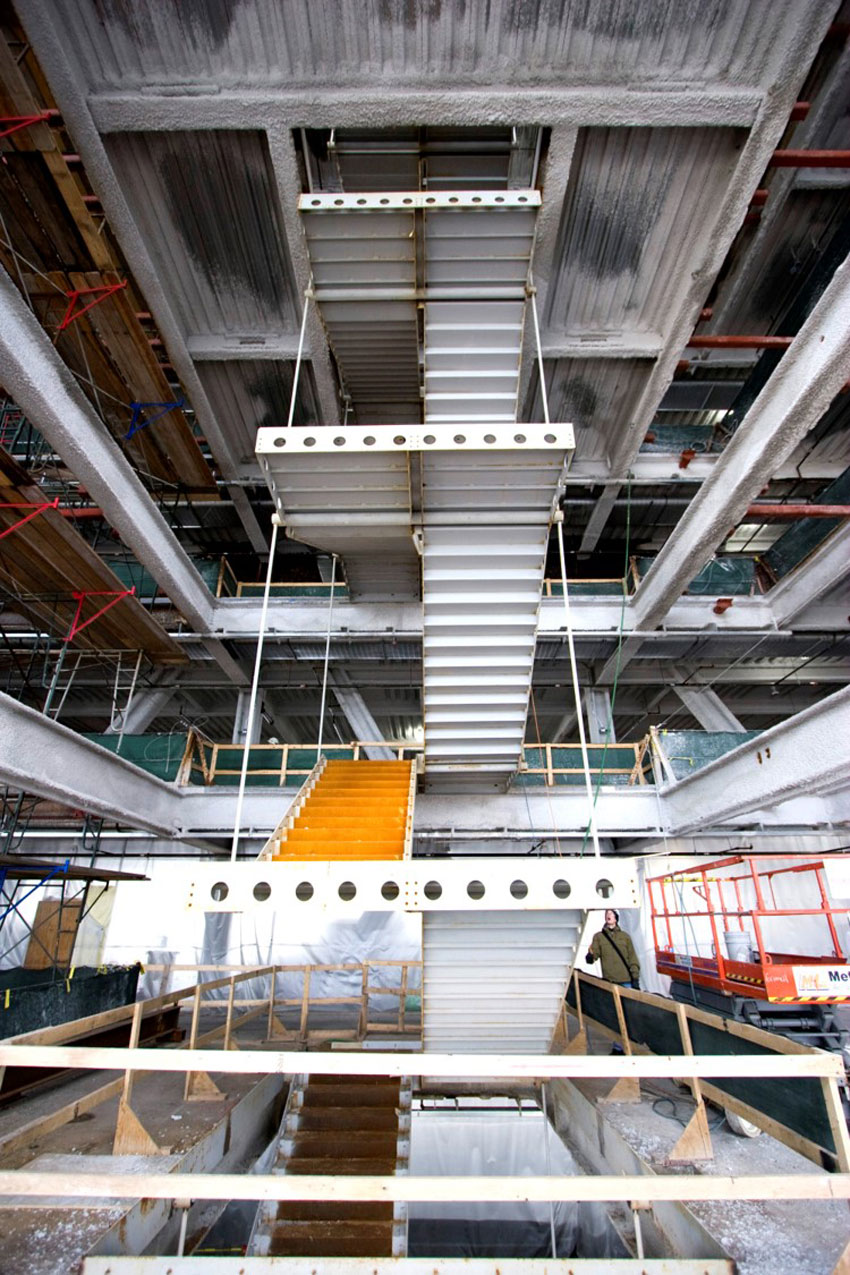
Photo courtesy of MKA
Pictured is the interior steel stairway hanging from the new construction above Blue Cross Blue Shield’s vertical expansion.
“The ability to assemble and disassemble parts of the original steel frame, combined with the lightweight solutions for the tower above, were all only possible in steel,” says Davies. “Being adaptable for future needs was a true sustainability win.”
Another example where early involvement with the steel fabricator led to significant savings is MKA’s design for the Frank Gehry Experience Music project in Seattle, which involved an extensive use of plate steel cut to customized shapes.
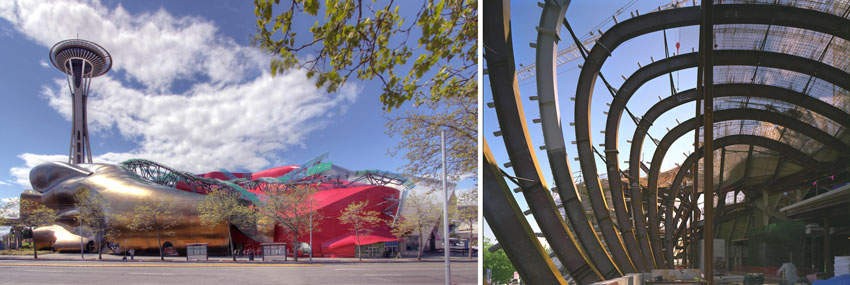
Photo courtesy of MKA
MKA’s design for the Frank Gehry Experience Music project features a steel rib cage, with each rib fabricated in a unique custom shape.
Looking for a way to minimize waste, the fabricator came up with an innovative solution utilizing software from the garment and shoe industry that optimized cutting different shapes out of a flat surface with minimal waste.
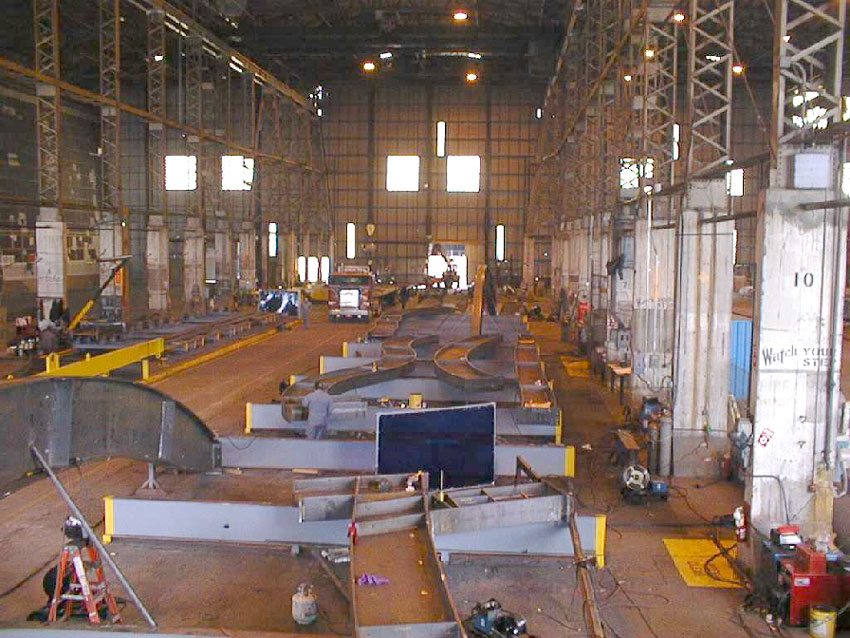
Photo courtesy of MKA
Here the ribs are being welded and laid out in the shop, capitalizing on a software tool from the garment industry.
“It was fascinating to see this kind of cross-industry evolution, and it helped to save both money and material,” relates Davies. “None of us are an expert at everything, and engaging those that know their part of the business best is almost always a winning combination.”
Clearly proving the benefits of the fabricator’s early involvement is the recent Mercy Heart and Vascular Center at the St. Vincent Medical Center project in Toledo, Ohio. The original design-bid-build project delivery model without fabricator input resulted in an over-budget project with a steel package valued at $2.8 million for 910 tons of structural steel. After transitioning to a design-build model that included the steel fabricator on the project team, the steel package was reduced to 772 tons at a cost of $2.35 million, with no change to the owner’s building program.
The global warming impacts associated with the reduction in tonnage fell from 1,056 tons of CO2 equivalent to 895 tons of CO2 equivalent, for a savings of 15 percent. “Additional savings were gained in the simplification of fabrication operations evidenced by a reduction in the per-ton cost of the fabricated steel,” report Cross. “It should be noted that the project schedule was also reduced by 16 weeks, from 52 weeks to 36 weeks.”
Conclusion
As the producer of a highly sustainable, multi-attribute building material, the structural steel industry has a proven track record and continues to stride toward further reducing the material’s environmental footprint.
Since 1990, the industry has posted an overall reduction of greenhouse gas and other emissions of 36 percent, while increasing the average strength of structural steel sections by 40 percent. Similarly, major improvements in mill technology have produced a reduction of the man-hours required to produce a ton of steel from 12 hours per ton in the 1970s to 0.6 man-hours per ton in 2016.
In conclusion, Davies states, “Steel lets us optimize building designs in very materially unique ways. Steel’s ductility when pushed past its yield point is ideal for seismic applications, its strength-to-weight ratio is superior, and its potential for preassembly and disassembly can lead to reuse in ways that other materials really can’t touch.”
Structural steel is truly a cradle-to-cradle, multi-attribute sustainable material.
Barbara Horwitz-Bennett is a trade press journalist who has covered the design and building industry for the past 17 years. She contributes regularly to a number of leading architectural publications.
![]() |
AISC is the leading advocate and trusted resource for American structural steel. Grounded in unsurpassed science, quality, and craftsmanship, our vibrant industry is ever-advancing and ever-improving. That’s why for nearly 100 years, AISC has proudly innovated with breakthrough research and applied technology for fabricators, engineers, architects, and the construction industry. We set the standard so you can focus on delivering visionary projects of the highest quality for your clients. As we move into our next 100 years, we’ll continue to advocate for, champion, and strengthen this vital industry. solutions@aisc.org. |