Acoustical Control in Buildings
Mitigating sound transfer between spaces through design, details, and insulating materials
Continuing Education
Use the following learning objectives to focus your study while reading this month’s Continuing Education article.
Learning Objectives - After reading this article, you will be able to:
- Analyze the background of acoustics and the fundamentals of sound as they apply to sound transfer between spaces.
- Examine the science and emerging standards for limiting background noise in green schools, hospitals, and other buildings.
- Assess the contribution that good acoustical design makes toward improved indoor environmental quality in green and sustainable building design.
- Identify the elements that make up a holistic approach toward acoustical design and beneficial sound control.
Sound is present in all buildings and affects people in a space just as other types of energy, such as heat and light, do. Controlling all aspects of sound entering or leaving a room or building space is the essence of good acoustical design. Unwanted sound that is allowed to enter from outside of a building can be distracting or even harmful to people inside. Sound flowing between rooms or spaces in a building in all directions (i.e. through walls, floors, ceilings, etc.) can create similar negative indoor experiences. All of these aspects of sound in a building are important to a successful design much the same way designing a building to use desirable light while controlling undesirable glare is important. By taking a holistic approach to acoustical design as an integral part of architectural and interior design, a comprehensive, successful result can be achieved. Controlling the transfer of unwanted sound between spaces reduces noise, improves the indoor environment, contributes to sustainable design, and is ultimately good for the people who use the building.
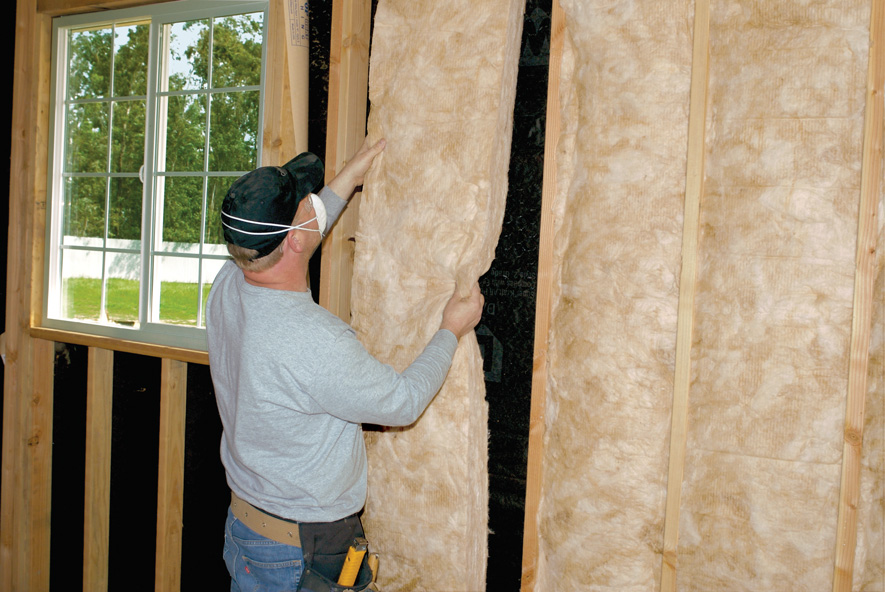
Photo courtesy of CertainTeed Insulation
Sound control in buildings involves attention to details, particularly in light-weight framed walls that use acoustical insulation to achieve desired results.
FUNDAMENTALS OF SOUND
Sound is energy, and like all energy, it radiates outward from a source. It moves the same way that heat energy and light energy do by traveling through air, solids, and other materials. While there are many beneficial sounds that we want to hear as they travel through space, such as speech in a classroom or music at a concert, there are other sounds that we don’t want to hear, which we call noise. This unwanted or interfering sound in building spaces can come from a variety of sources both within and outside of a building, such as outdoor traffic, activity in an adjacent room, or mechanical equipment.
A certain amount of background noise is certainly commonplace and even acceptable. However, excessive background noise can seriously degrade the ability of people to communicate, overshadow preferred sounds, or prevent audible signals from being heard.
The scientific study of sound and noise has not only looked at the physics of sound, but evolved into the field of acoustics, which looks at sound in all its forms. Architectural acoustics in particular is specifically related to the interactions of sound both within and between architectural spaces and the impacts on people in those spaces. As such, a significant body of knowledge is available on how to deal with sound in human environments as part of the field we know as acoustical design.
Sound Generation
Sound can be generated from single or multiple sources but is typically characterized by three characteristics.
- Frequency (Pitch): Sound has been observed to travel in oscillating waves that radiate out from the source. Those waves are measured in terms of the cycle of the sine-shaped wave that oscillates from neutral, down to negative, up to neutral, further up to positive, and finally back to neutral. The time it takes to complete that one cycle of a sound wave is measured in hertz (Hz). One Hz is equal to a frequency of one cycle per second. The human ear can typically hear frequencies or sound “pitch” between about 20 Hz (very low-pitched, bass sounds) up to around 20,000 Hz (very high-pitched, piercing sounds) if the person is fairly young; older adults may hear frequencies up to about 15,000 Hz. Sound exists above and below this range, but our ears don’t hear it, unlike some animals, such as dogs or bats, that do indeed hear very high-pitched sounds (over 20,000 Hz) or elephants that can hear lower sounds (below 20 Hz).
Image courtesy of CertainTeed Insulation
Sound is characterized by its frequency (pitch), wavelength, and amplitude (volume).
- Wavelength: The wavelength of a sound wave is the distance between the start and end of a sound wave cycle or the distance between two successive sound wave pressure peaks. Numerically, it is equal to the speed of sound in the material, such as air divided by the frequency of the sound wave. For example, the wavelength of a 100 Hz tone at room temperature is 1,130 feet/second (the speed of sound in air) divided by 100 Hz (a fairly low-pitched sound), which is equal to a wavelength of 11.3 feet. By comparison, dividing the speed of sound by a much higher pitch of say 10,000 Hz would produce a much shorter wavelength of .113 feet or a little more than an inch.
- Amplitude (Loudness): The amplitude of a sound wave is visually its height from bottom to top, but is in fact indicative of the strength of the energy in that wave. It is measured in decibels (dB), such that the higher the dB rating, the more energy, and hence the louder the sound that we hear. The common range begins at 0 dB (threshold of hearing) to around 130 dB (threshold of pain), with human speech being in the middle around 50 to 65 dB. The most widely used sound level filter is the A scale, expressed as dBA. Using this filter, a sound level meter is less sensitive to very high and very low frequencies.
Sound waves can travel through any media, such as air, water, wood, masonry, metal, etc. Depending on the media through which it travels, sound is either considered airborne or structure-borne. Airborne sound radiates from a source and travels through the air. The sound of traffic passing our homes, the sound of music or voices from the next room or office, the noise from low flying aircraft—all travel to our ears as airborne sound. Structure-borne sound travels through solid materials usually in direct mechanical contact with the sound source or from an impact on that material. Examples are footsteps or objects falling on the floor upstairs, a knock at the door, or vibration from loud speakers on the floor. All structure-borne sound must eventually become airborne sound in order for us to hear it. We can only feel structure-borne sound as vibrations in a material. In most noise control situations, both airborne and structure-borne sound must be considered.
Sound Transmission
When sound travels through air and strikes a surface, such as a wall, floor, or ceiling, it behaves like other energy. Some of the sound energy is reflected back off of the surface, some is absorbed into the material or construction assembly, and some is transmitted directly through it. This is directly akin to light, which can be reflected off a glossy surface, absorbed into a dark surface, or transmitted through a translucent material. Similarly, different amounts of sound energy can be reflected, absorbed, or transmitted when striking different surfaces, materials, and assemblies in buildings. The following is a summary of terms and measures relating to principles of controlling sound transmission between spaces.
- Transmission Loss (TL) is a fundamental measurement of the ability of a material or building assembly to block or reduce the amount of sound passing through it. It is measured in decibels at different frequencies to determine how much sound transmission is lost at each measured frequency. Generally speaking, a TL of 10 means that the sound is 10 dB quieter on the listening side compared to the sound-originating side.
- Sound Transmission Class (STC) is determined by ASTM E90 test methods to measure airborne transmission loss specifically in interior walls and ceiling/floor assemblies. A higher STC rating means that more airborne sound is blocked by the component or assembly. Lower STC ratings mean that more sound passes through the components or assemblies, adding to the background noise level in the space. It should be noted that, contrary to the popular notion that sound passes through a structure, such is not the case. Sound generated on one side of a wall will energize the wall structure and set it in motion, much like a diaphragm. The wall itself becomes the transmitter of the sound energy, which can be heard on the opposite side of the wall by the listener. Hence, the ASTM test methods used to determine STC ratings have focused on this direct transmission process, although this testing has changed over the years, meaning that STC results posted before 1999 may not produce the same results today.
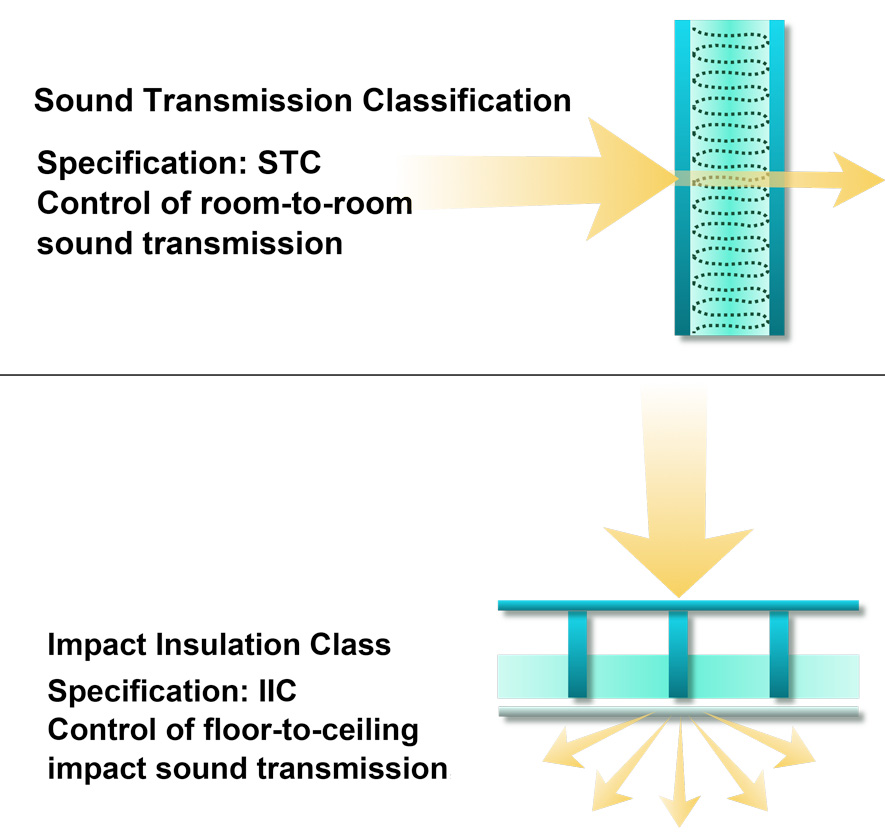
Images courtesy of CertainTeed Insulation
The sound transmission class (STC) is a measure of airborne sound transmission loss, while the impact insulation class (IIC) is a measure of structure-borne sound transmission loss.
Currently, the STC number is derived from sound values tested at 16 standard frequencies from 125 Hz to 4,000 Hz. These transmission-loss values are then plotted on a sound pressure level graph, and the resulting curve is compared to a standard reference contour. Acoustical engineers fit these values to the appropriate transmission loss curve to determine a final STC rating. The STC measurement is accurate for speech sounds but less so for amplified music, mechanical equipment noise, transportation noise, or any sound with substantial low-frequency energy below 125 Hz. Nonetheless, since this is fundamentally a measure of TL, the derived STC number generally reflects how many decibels quieter the receiving side of a wall is compared to the sound-originating side (e.g. STC 45 means a reduction of 45 dbA). In terms of people’s perception of sound, a general rule of thumb is that each 10-point increase in STC value will decrease the perceived noise by one half of the starting point.
- Outdoor Indoor Transmission Class (OITC) is a standard method used to rate the amount of transmission loss in an exterior wall setting, such as determining how much traffic noise an exterior wall can stop. The OITC covers a lower or broader frequency range from 80 Hz to 4,000 Hz, where most aircraft, rail, and vehicular traffic sounds would be found.
- Impact Insulation Class (IIC) is a measure of the ability of a floor-ceiling assembly to absorb or deflect sound from impacts (such as people walking or objects dropping) and keep it from being transmitted to the space below. It is not a measure of airborne sound but rather of structure-borne sound. The IIC rating is determined by ASTM Standard E989: Standard Classification for Determination of Impact Insulation Class (IIC). Test data obtained in accordance with ASTM E492: Standard Test Method for Laboratory Measurement of Impact Sound Transmission Through Floor/Ceiling Assemblies Using the Tapping Machine, is used to determine the IIC rating of a floor.
- Ceiling Attenuation Class (CAC) is a measure for rating the performance of a ceiling system as a barrier to airborne sound transmission through a common plenum between adjacent closed spaces, such as offices. CAC for ceilings is similar to an STC rating for walls, but is specific to suspended ceiling conditions where a dividing wall is constructed only to the ceiling height, thus allowing sound to pass through a plenum space above the ceiling. As an ASTM E1414 tested measurement, it is a ‘two pass’ test in that the sound is looked at as it transmits up through the ceiling in one space, across the ceiling plenum, and back down through the ceiling in an adjacent space. A higher CAC rating means that less sound is transferred between spaces. A ceiling system with a CAC below 25 is considered low performance, whereas one with CAC above 35 is high performance.
SOUND AND INDOOR ENVIRONMENTAL QUALITY
With an understanding of the principles of sound generation and sound transmission, we can focus on the impact of sound and noise on the indoor environment. We have all likely experienced situations where unwanted background noise has interfered with normal activities in a room or space. The response, if we are talking, is typically to speak louder. In fact, it is generally accepted that most people would need to speak at least 15 decibels (dBA) louder than the background noise level in order to be heard at all. This observation and others has led to the development of national standards, such as ANSI Standard S12.60: Acoustical Performance Criteria, Design Requirements and Guidelines for Schools, which establishes some very stringent thresholds for background noise. Specifically, for core learning spaces of 20,000 cubic feet or less, the one-hour steady-state background noise levels should not exceed 35 dB, while those more than 20,000 cubic feet should not exceed 40 dB. This is the same low level of sound that one would experience in a quiet office.
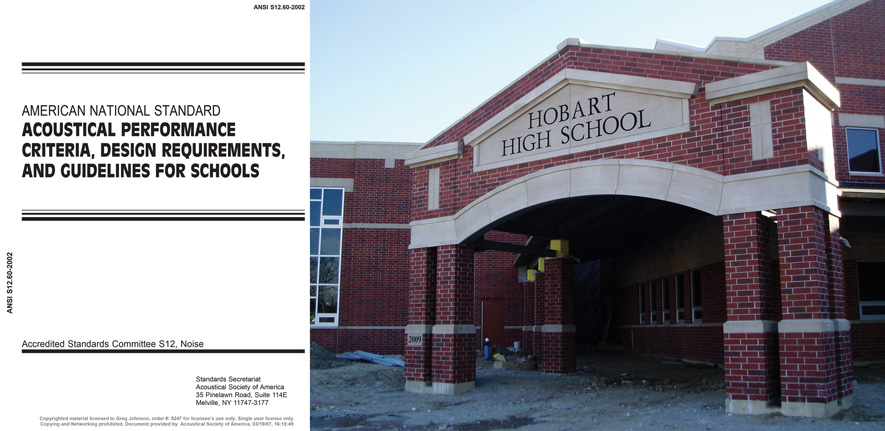
Images courtesy of CertainTeed Insulation
ANSI Standard S12.60 defines Acoustical Performance Criteria, Design Requirements, and Guidelines for Schools and is the basis for LEED for Schools acoustical prerequisites and credits.
ANSI S12.60 has also been recognized by the U.S. Green Building Council (USGBC) as a basis for performance in the LEED for Schools program with specific criteria in two cases. First, there is a mandated prerequisite for minimum acoustic performance in the Indoor Environmental Quality (EQ) portion of LEED. The stated intent of this prerequisite is, “To provide classrooms that are quiet so that teachers can speak to the class without straining their voices and students can effectively communicate with each other and the teacher.” The LEED prerequisite requirements follow the same ANSI thresholds for size and dBA levels of background noise, although it is only stated to address HVAC equipment noise.
There is also an additional IEQ credit for Enhanced Acoustic Performance with a stated intent, “To provide classrooms that facilitate better teacher-to-student and student-to-student communications through effective acoustical design.” In educational settings under ANSI Standard S12.60-2002, single or composite wall assemblies should provide STC ratings whenever separating a core learning space (e.g. a classroom) from other specific adjacent spaces as follows:
- STC-45 if the adjacent space is a corridor, staircase, office or conference room;
- STC-50 if the adjacent space is another core learning space, speech clinic, health-care room, or outdoors;
- STC-53 if the adjacent space is a restroom;
- And STC-60 if the adjacent space is a music room, mechanical equipment room, cafeteria, gymnasium, or indoor swimming pool.
- Classroom doors should be rated as STC-30 or more, and music room doors as STC-40 or more. Commonly, entry doors located across a corridor are staggered to minimize noise transmission from one room to another across the hallway.
It should be noted that open-plan classroom designs will not meet the requirements of this standard since there is nothing to impede the sound transmission from one space to another. In addition, STC ratings ranging from 45-60 are also outlined in the ANSI standard for assemblies separating non-classroom spaces from adjacent spaces.
Since classrooms can also be above or below each other, ANSI Standard S12.60 also identifies specific impact insulation class (IIC) ratings and recommendations for classroom learning spaces, including the following:
- IIC ratings for floor-ceiling assemblies above core learning spaces should be at least IIC-45 and preferably IIC-50 as measured on floors without carpeting.
- New construction, a gymnasium, dance studio, or other spaces with high floor impact activities shall not be located above core learning spaces.
- In existing facilities, IIC-65-70 (depending on the volume of the space below) is recommended if gymnasia, dance studios, or other spaces with high floor impact activities are located above core learning spaces.
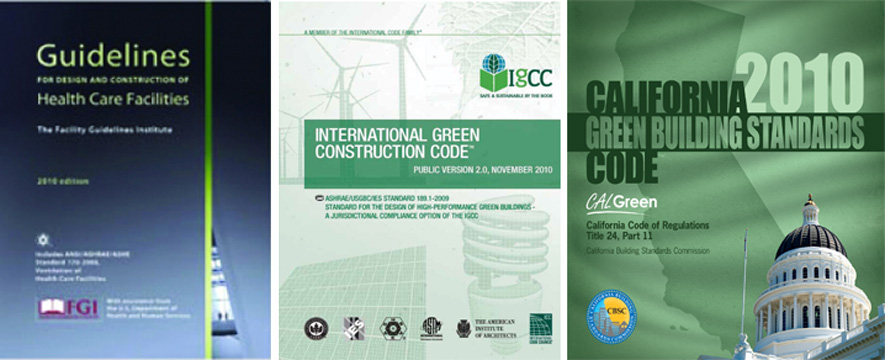
Images courtesy of CertainTeed Insulation
An increasing number of codes, standards, and guidelines are addressing acoustical performance as a matter of public health, safety, and welfare.
Standards like this are becoming more common for other building types, too. Hospitals and health-care facilities are recognized in LEED v4 with criteria for sound control as part of the Indoor Environmental Quality portion. Additionally, the 2010 FGI Guidelines for Design and Construction of Healthcare Facilities, a recognized standard for minimum space, architectural detail, and surface and furnishing requirements in healthcare facilities, also address acoustics and speech privacy. They indicate that background sound generated by building systems and sound isolation need to be considered for occupied spaces in order to reduce noise produced annoyance. They reference the Sound and Vibration (SV) Design Guidelines for Hospitals and Healthcare facilities for compliance. Related to that, Health Insurance Portability and Accountability Act (HIPAA) regulations require speech privacy and room noise levels not to exceed specific compliance requirements.
When it comes to residential buildings of all types, there are a number of standards for privacy and sound control. This is true particularly in multifamily buildings, hotels, and other similar occupancies. The specific criteria look at STC and IIC ratings between adjacent spaces with predictably higher ratings needed between bedrooms and active areas. Suitable ratings for office and workplace spaces have been investigated and developed. The recommended STC ratings for a variety of adjacencies are published in Architectural Graphic Standards and promulgated by the North American Insulation Manufacturers Association (NAIMA) headquartered in Alexandria, Virginia.
Green Building Codes, such as the International Green Construction Code (IgCC) and the California Green Building Standard (CalGreen), similarly address acoustic control in all types of buildings. Many of these standards and requirements are responding to independent research conducted in a variety of university and clinical settings to determine the relative effects of noise on people in indoor environments. The findings have been convincing enough to determine that background noise can contribute to poorer performance in schools, longer patient recovery times in health-care settings, and detrimental health effects to employees in any setting. As a matter of public health, safety, and welfare, then, acoustics is increasingly being viewed as a significant component.
SOUND MITIGATION IN EXTERIOR AND INTERIOR WALLS
With an understanding of the principles and requirements of sound control, we can turn our attention to some ways to achieve the needed acoustic performance. Fundamentally, there are only three basic ways to attenuate or reduce sound. First, we can address the source by replacing the source sound with a quieter one when that is in our control, such as locating quieter rooms away from noisier ones. Second, we can address the listener’s location by blocking sound from entering the space with solid, heavy material that resists the transmission of airborne sound waves. Third, we can consider the path that sound travels from the source to the receiver and not only seek to resist transmission, but use lighter, porous materials that can absorb or soak up the sound waves. These three ways to control sound are the basis for the strategies discussed in the following paragraphs.
When looking at walls, it is all about the design and details of the wall assembly. Sound striking a surface of a wall causes that surface to vibrate, much like the diaphragm of a drum. The more massive the wall, the less the amplitude of vibration of the wall. This results in less noise being transmitted to the room on the other side of the wall. In cases of exterior walls in large commercial buildings where OITC is being addressed, this sheer mass approach may be a viable one. Since most new exterior walls are filled with insulation and fairly well-sealed for thermal performance, the good news is that those same measures should help notably with acoustical performance. If heat energy flow can be restricted in a wall then sound likely will be, too.
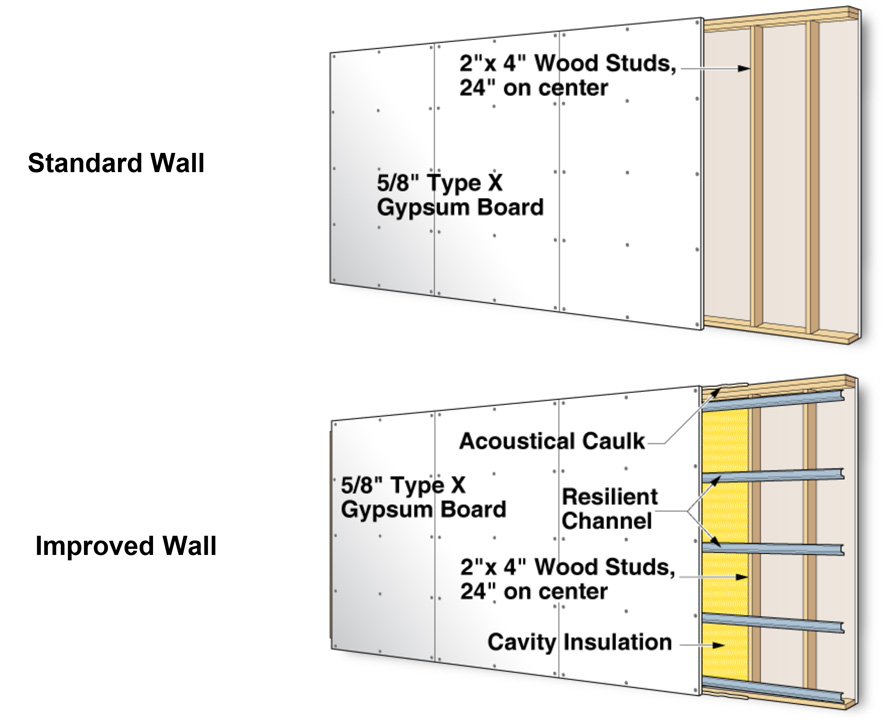
Images courtesy of CertainTeed Insulation
Multiple strategies exist to improve on the acoustical rating of a standard framed wall (STC 30) compared to one with acoustical insulation and resilient separation of gypsum board from the studs (STC 50).
For interior walls and partitions, it is rarely practical to rely on sheer mass to reduce the transmission of noise. Rather, lighter-weight framed walls are more common. In a conventional framed interior partition (e.g. ½-inch gypsum wallboard on either side of 2-by-4 wood studs on 16- or 24-inch centers) sound vibration is readily transmitted through the gypsum board, the studs, and the air space between the studs to the opposite side of the wall where it is heard as noise. In this case, the sound reducing property of the air space is negated by the studs, which form a direct structural connection between the two wall surfaces and allow the sound to pass through. Such a wall would likely test out with an STC rating of around 29. In practice, a wall system in an office setting with an STC 35 or less is considered low performance, whereas one with an STC 55 or higher is considered high performance.
In order to improve the amount of transmission loss and the corresponding increase in STC ratings in lightweight walls, several strategies are common. The use of light-gauge steel studs at 24 inches on center, instead of wood studs at 16 inches on center, can help increase the values in some scenarios since the thin steel stud may provide less of a connection between the two wall faces and allow less sound energy to be transmitted. One of the most cost effective and straight forward things to do is install 3½-inch-thick fiberglass acoustical insulation in the stud cavity of either wood or steel framed assemblies. Taking the effect of the insulation into account with ½-inch gypsum board on both sides, the performance improves in a wood framed wall to STC 39 and in a steel stud framed wall to STC 48. To improve the wood framed assembly, resilient channels can be used to isolate the materials and raise the STC rating. Such resilient channels, of which there are several types and designs available, can be installed across the face of the studs before the gypsum board is installed, thus eliminating direct mechanical connection of the gypsum wallboard to the studs. With the combination of insulation, ½-inch gypsum board on resilient channels (one side) spaced at 24 inches vertically, the STC rating of a wood framed wall can be increased to STC 48.
Adding additional layers of gypsum board on one or both sides is also effective since it provides some increased mass, which can reflect more sound and decrease the amplitude of vibration, thus reducing the transmitted noise level on the other side of the wall. Using two layers of ½-inch gypsum board on wood framed walls with insulation brings the STC rating up notably from 39 to 55 and up to STC 57 if resilient channels are used. In steel stud framed walls with two layers of ½-inch gypsum board and insulation, the performance increases from STC 49 up to STC 55. If 5⁄8-inch gypsum board were used in two layers on each side, instead of ½-inch, the performance can increase by an additional STC rating level of 2 to 4.
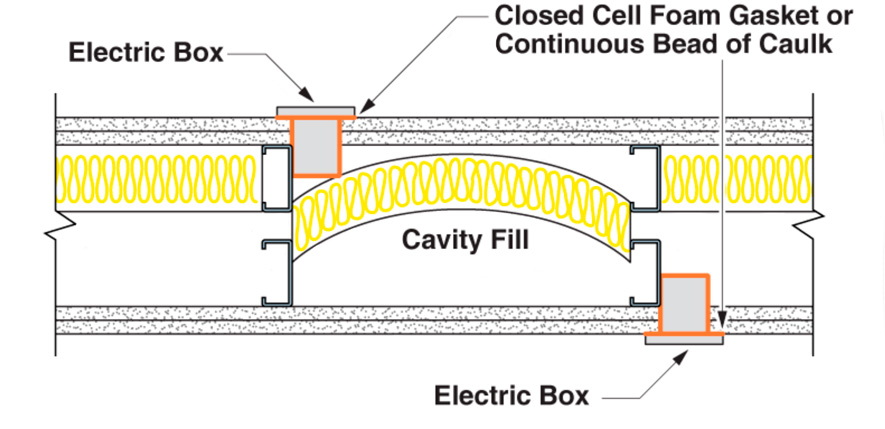
Image courtesy of CertainTeed Insulation
More creative strategies, such as double-stud separated framing coupled with insulation, double layers of gypsum board, and sealing around penetrations that are separated, can produce superior results in the range of STC 60 or better.
Alternatives to adding more gypsum board exist by framing the walls a bit more creatively. For example, while a typical 2-by-4 wall would commonly have a 2-by-4 top and sill plate, using 2-by-6 plates allows the studs to be staggered on the plates and isolate the gypsum board on each side at every other stud. This would raise the STC rating, with insulation, from STC 39 to STC 45, or to STC 48 if 5⁄8-inch gypsum board were used. If still higher performance is needed, then it is common to separate the staggered studs into a double-stud wall construction. In this case, two rows of studs are installed with a gap between the rows so none of the studs, top plates or sill plates touch each other. This breaks the mechanical connection between the two wall surfaces, completely interrupting the sound transmission. Then a double layer of fiberglass sound absorbing insulation can be installed in the wall cavity and be fully effective since it is no longer compromised by the studs. The STC rating of this type of assembly can range from STC 58 with one layer of ½-inch gypsum board on each side of wood studs up to STC 67 with two layers of 5⁄8-inch gypsum board on each side. Comparable performance can be achieved in steel stud framed walls.
When it comes to selecting the acoustical insulation to use in assemblies like these, it is incorrect to assume that higher-density insulation within the wall system provides better sound transmission loss. Comparative tests conducted at nationally recognized acoustical laboratories have shown that increasing the density of the insulation while maintaining a constant thickness does not have a significant effect on the STC rating of the construction. It is also incorrect to assume that heavy insulation in the stud cavities increases the STC because it adds weight to the wall. Actually, to increase the STC of a wall by adding weight, the weight must be added to the faces of the wall, not its core. For these reasons, mineral or rock wool insulation has not been shown to perform better than low-density fiberglass insulation when comparing identical thicknesses. These same tests show that insulation thickness within the wall cavity is the most important property, and that complete filling of the cavity between wall surfaces provides the best wall performance.
Beyond this isolation of surfaces, it is appropriate to consider all of the gaps and openings that can exist around framing and other wall materials. Avoiding direct pathways through penetrations of the wall is important, including things like electrical outlets and other things that are commonly run in stud-space cavities. Using an acoustical sealant will help fill the gaps around openings and at connections and prevent sound from being transmitted through these wall penetrations. Acoustic sealants commonly include silicone, latex, or acrylic-based formulations acoustically engineered to block sound with non-drying and non-hardening characteristics that allow it to retain its acoustic properties over time. Acoustic sealant is available for interior and exterior applications on a variety of different surfaces, including gaps between wall stud plates and subfloor, openings around electrical outlets and boxes, air ducts and boots, doors, windows, and other wall and floor penetrations or gaps. Sealant can contribute to a reduction in perceived noise levels through the walls on the order of 2 to 3 dB, which is enough to be noticeable.
SOUND MITIGATION IN CEILINGS AND FLOORS
At the basic level, airborne sound can be attenuated in ceilings in a manner similar to walls by adding sound absorptive insulation in ceiling and floor systems, adding resilient channels, and additional gypsum board. In framed construction with floor/ceiling joists, the techniques and performance are similar to wall construction techniques. For suspended ceilings, some insulation is designed specifically to be used in the plenum space above to economically improve both noise control and possibly thermal performance in new or existing ceiling systems. When placed on top of a suspended ceiling at a floor-to-ceiling partition, this type of insulation has been shown to help reduce the transfer of sound over the partition between rooms. As such, it can improve the ceiling attenuation class (CAC) rating on the order of 4–10 points.
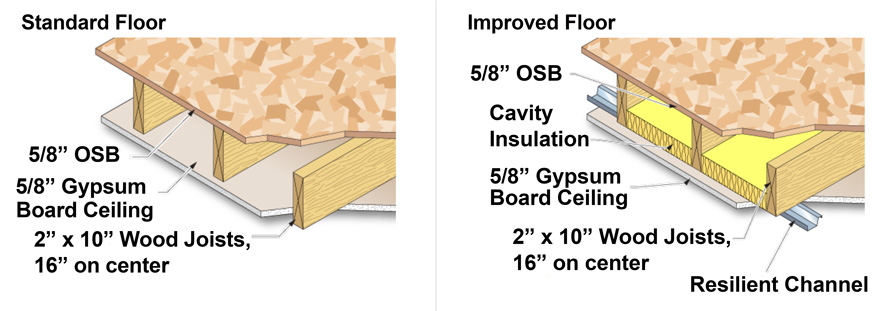
Images courtesy of CertainTeed Insulation
In multi-story buildings, the ceiling/floor assembly needs to address both IIC and STC equally with strategies that reduce both airborne and structure-borne sound.
In multi-story buildings, the ceilings are also a floor for the story above. The measures described, along with appropriate sealing, will still work very effectively in these cases for airborne sound. However, the sound transmitted due to impacts need to be addressed differently. In this case, a material is needed to reduce the transmission of sound from people walking, dropping things, or operational activities. In some cases, the flooring itself can help reduce sound, such as carpeting or cushioned vinyl flooring. However, that typically isn’t enough to address areas with a fair bit of activity. Rather, the most effective approach is to install a continuous layer of material with a high impact insulation class (IIC) rating between the subfloor and the finish flooring. Floor matting of this type is commonly composed of closed cell, extruded polyethylene foam, or ethylene propylene diene monomer (EPDM). It is acoustically engineered to reduce structure-borne sound by isolating it to the room in which it is created. By placing it between the subfloor and then installing a plywood or gypsum concrete layer on top, it creates a continuous layer to isolate impacts, dissipating some energy and reflecting the remainder back into the space above. The installed plywood or gypsum concrete layer “floats” on top of the floor mat, thus providing a rigid surface for the finished flooring.
To increase both the STC and IIC ratings of a floor/ceiling construction, fiberglass insulation should be installed in the joist cavity, with a resilient ceiling system below the joists for best results. The IIC rating of a floor/ceiling assembly should be equal to or better than its STC rating to achieve equal performance in controlling both airborne and structure-borne sound. As with partition and wall construction, penetrations in sound-rated floor/ceiling assemblies should be caulked airtight. Lighting fixtures and other items should be surface mounted, not recessed to avoid the penetration of the ceiling surface.
SOUND CONTROL IN MECHANICAL SYSTEMS
HVAC systems typically require ductwork to move air within the building for space conditioning or ventilation or both. From an acoustical standpoint, ductwork is a penetration in a wall or ceiling that has the potential to bring noise into a space. If not acoustically treated, noise from heating, ventilating, and air-conditioning equipment, such as fans and motors, can travel through the ductwork from room to room in a building. The velocity of the air in the duct system can cause noise-producing turbulence, while also causing turning vanes, dampers, and other elements inside the ducts, grilles, and diffusers to whistle or rattle. HVAC ductwork can also act as a “speaking tube,” acting as a means to carry conversations from one room or office into other spaces.
To reduce the noise from HVAC equipment, a good guideline is “lower and slower”: lower volumes of air moving through the system with fans and blowers operating at a slower speed will lower the source of the noise. Central air equipment should also be acoustically isolated from spaces where airborne noise would be objectionable. Equipment should be mounted on vibration isolators to avoid transmission of structure-borne noise. Sound traps or baffles will help to attenuate equipment noise in adjacent ductwork.
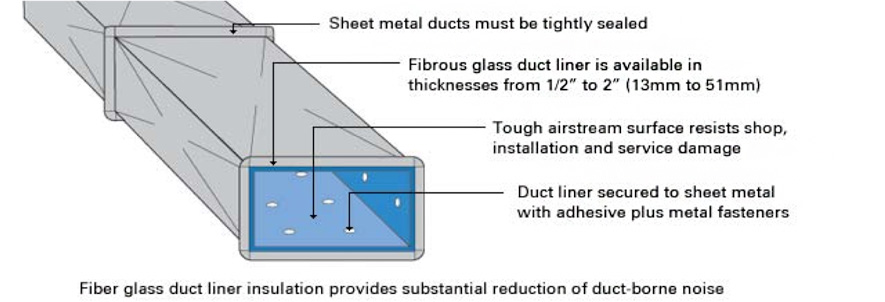
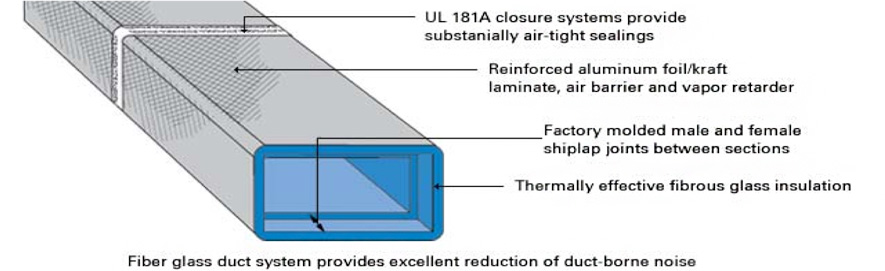
Images courtesy of CertainTeed Insulation
Fiberglass duct liner insulation provides substantial reduction of duct-borne noise (top), while fiberglass duct systems can provide superior sound deadening (bottom).
When it comes to the ductwork itself, recognizing that it can be a source of noise as well as a transmitter of it is important. Sheet metal ductwork without insulation can produce popping and banging noises due to expansion and contraction caused by changes in air temperature. The air turbulence in ductwork can also be problematic. Most of the ductwork noise problems can be solved with fiberglass duct insulation in either of two forms. First, a specifically designed fiberglass duct liner can be installed inside sheet metal ductwork to attenuate air rush and central equipment noise. This fiberglass duct liner has the added benefit of helping to control heat loss or gain through duct walls. Second, fiberglass duct board can be used to fabricate the ductwork. This approach combines acoustical/thermal insulation with a reinforced foil-kraft air barrier/vapor retarder, allowing a complete air-duct system to be created. Either of these approaches have been found to be effective ways to control sound in HVAC systems. Fiber glass ducts wraps, used as thermal insulation on the outside of sheet metal ducts, provide little acoustical benefit except by muffling the popping and banging noises when ducts undergo temperature changes.
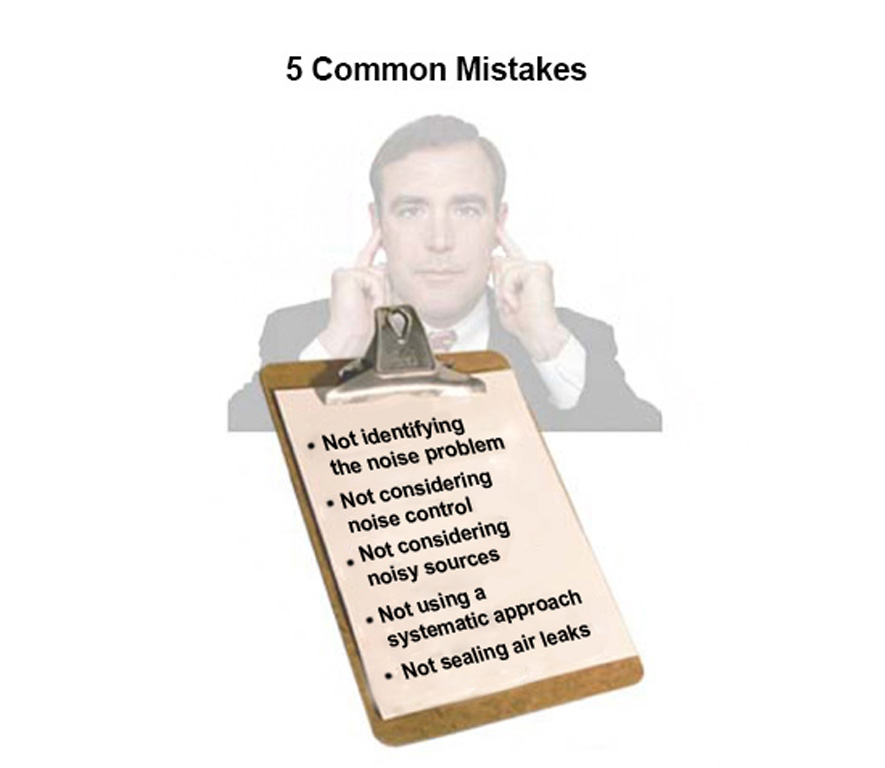
Image courtesy of CertainTeed Insulation
By taking a proactive approach to acoustical design in buildings, common mistakes and problems can be avoided.
CONCLUSION
Architects and designers are well served when they take time in the design process to consider acoustical needs. By taking a proactive approach, there are a few simple steps to avoid common mistakes and help assure a successful outcome. First, recognize that the building may have a noise problem if acoustics aren’t addressed properly. In general, if communication is difficult because of noise, a problem exists. Second, consider noise control from the outset. Although a source of noise can be treated after construction is complete, it’s generally twice as expensive and half as effective compared with designing proper noise control in the first place. Third, conduct a detailed study of noisy equipment or other problem sources. In doing so, consider if the frequency range is typical or falls in the more easily transmitted lower ranges. Fourth, use a systems approach to noise control. A common waste of noise control dollars is the failure to consider all possible solutions and noise paths. To treat one noise source and not consider all possible noise sources could lead to unacceptable noise levels when a project is completed. The same is true if only one path of noise transmission is considered. All airborne and structure-borne noise paths must be studied. Fifth, seal air leaks. Sound always takes the easiest path around or through a barrier. Construction gaps or air leaks are by far the easiest way for sound to pass from one space to another. Combining this approach with the principles and strategies discussed, it is entirely possible to achieve a healthy, sustainable, indoor environment for buildings of all types.
Peter J. Arsenault, FAIA, NCARB, LEED AP is an architect and green building consultant who has authored more than 120 continuing education and technical publications as part of a nationwide practice. www.linkedin.com/in/pjaarch
![]() |
CertainTeed Insulation |